Is Longevity Genetic? 11 Studies That Reveal Your Control Over Aging
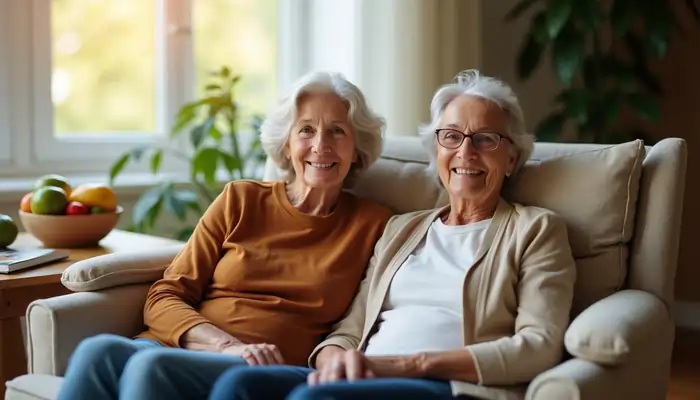
Research shows that siblings and children of people who live longer stay healthier and outlive their peers. Scientists have made substantial progress in understanding longevity genes and have discovered around 57 gene loci connected to lifespan. Life expectancy has shown remarkable improvement – take Italy as an example, where it jumped from 29 years in 1861 to 82 years in 2011.
In this piece, we’ll look at 11 groundbreaking scientific studies that show the complex relationship between aging genetics and actual lifespan and try to answer well known question ” Is Longevity Genetic? ” . The sort of thing I love about this research ranges from twin studies to innovative technology in epigenetics. These findings reveal whether life expectancy comes from our genes and what that means to your health experience.
The Danish Twin Study
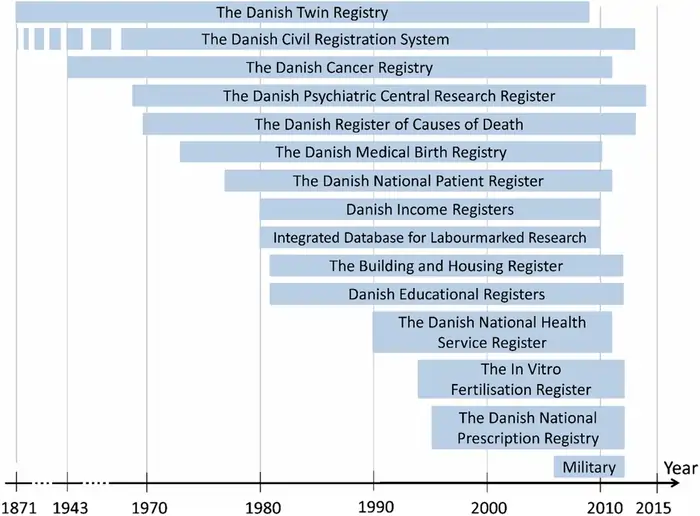
Image Source: Cambridge University Press
“The heritability of longevity was estimated to be 0.26 for males and 0.23 for females. The small sex-difference was caused by a greater impact of non-shared environmental factors in the females.”
— Axel Skytthe, Researcher at the Danish Twin Registry, University of Southern Denmark
The Danish Twin Registry ranks among the world’s best resources to understand answer on question ” is longevity genetic ” and the genetic foundations of human longevity. This 1950s old registry tracked twins born between 1870 and 1910, and by 2019 it included over 175,000 twins from 140 birth cohorts [1]. The registry’s size and age make it the oldest nationwide twin register and one of the largest globally.
Danish Twin Study background
Twin studies give scientists a natural experiment to separate genetic and environmental influences on aging. The Danish Twin Study focused on same-sex twin pairs who lived past age 15, which created robust data to analyze longevity patterns [2]. The final follow-up in May 1994 showed remarkable completion rates – all but one of these subjects had passed away, which provided reliable data [2].
Scientists used questionnaires about physical similarities to determine zygosity, and this method proved 95% accurate when checked against biological markers [1]. The team also accessed death records through the Danish Central Person Register and public registries to verify data accuracy [3].
Key findings on heritability
Is Longevity Genetic? – longevity has only moderate heritability. Scientists estimated heritability at 0.26 for males and 0.23 for females [2], which suggests genes account for about a quarter of human lifespan variation.
The study showed these key points:
- Similar twins had matching death ages, while fraternal twins showed no such pattern [4]
- Twin similarities pointed to non-additive genetic effects rather than additive genetic influences [3]
- Environmental factors that created differences between twins mattered more than their shared family environment [2]
McGue and team found something fascinating in the largest longitudinal study of Aging Danish Twins. When they looked at different twin pairs, especially similar twins, environmental factors explained outcome differences better than genetics [5].
Implications for longevity genetics
” Is Longevity Genetic? ” question is challenged by these results as well as a basic ideas about longevity inheritance. Even with modest heritability, genetic influence stayed stable across different birth cohorts from 1870-1900. This suggests consistent genetic mechanisms despite major changes in living conditions during that time [3].
The research proved that environmental factors, especially those that make people different from each other, play the biggest role in determining lifespan. Yes, it is worth noting that shared family environment showed no effect on longevity [2].
The Danish Twin Study’s value comes from its complete population-based approach, minimal censoring, and long-term design. The findings match evolutionary theory, which predicts traits tied to evolutionary fitness should have low heritability [3].
You can learn more about this research through the National Institute on Aging (www.nia.nih.gov), the Danish Twin Registry (www.sdu.dk/dtr), and the Human Mortality Database (www.mortality.org).
FOXO3 Gene and Longevity
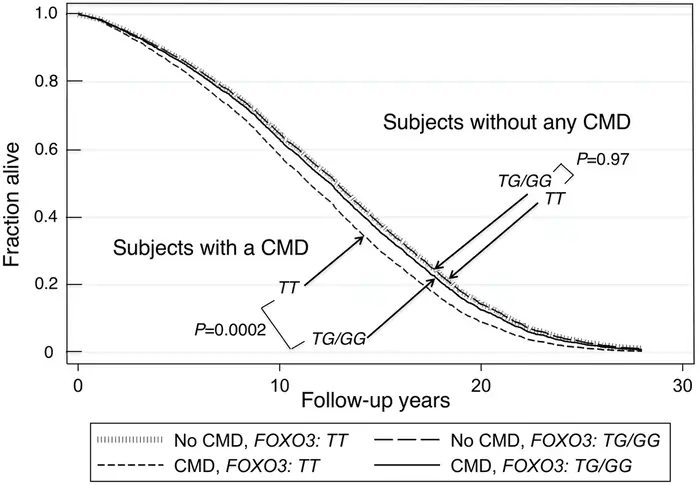
Image Source: Aging-US
FOXO3 stands out among the few genes consistently connected to human longevity. Scientists first discovered this fascinating gene by studying long-lived Japanese-American men in Hawaii. Research has since verified it as a key factor in longevity across populations worldwide [6].
FOXO3 gene function
FOXO3 is part of the “Forkhead box” family of transcription factors. These proteins work like genetic switches by attaching to specific DNA regions that turn genes on or off [7]. What makes FOXO3 special? It sits at the heart of multiple biological pathways that keep our cells healthy.
The gene has five structural domains, with a DNA binding domain that lets it work as a transcription factor [8]. Here’s what FOXO3 controls:
- Energy metabolism and insulin signaling
- Cellular stress responses
- Stem cell maintenance and renewal
- Autophagy (cellular “cleanup” processes)
- Inflammation control
You’ll find FOXO3 in species from worms to humans – this preservation through evolution shows just how vital it is for survival [9].
FOXO3 and lifespan extension
Scientists have found specific FOXO3 genetic variants (single nucleotide polymorphisms or SNPs) that link to longer life. The G-allele of rs2802292 stands out – people who have it are 1.9 times more likely to live past 95 than those with the common T-allele [9].
Scientists looked at 11 different studies and found that five FOXO3 SNPs showed clear connections to exceptional longevity [9]. These helpful variants seem to work by boosting FOXO3 expression, which turns up this protective gene’s activity [10].
Is Longevity Genetic? – Recent findings show another interesting effect: people with the FOXO3 G-allele keep longer telomeres as they age [9]. Since telomeres act like aging clocks in our cells, this protection might directly help people live longer.
Why FOXO3 matters in aging
FOXO3’s benefits go beyond just living longer – it affects how we age. People with good FOXO3 variants get less cardiovascular disease and cancer [8]. Their bodies also handle insulin better, which means lower diabetes risk [7].
Inside our cells, FOXO3 runs the cleanup system called autophagy, which removes damaged parts before they cause problems [8]. The way it controls inflammation matters too, since ongoing inflammation (“inflammaging”) leads to many age-related diseases [9].
The gene’s most impressive job might be protecting adult stem cells throughout the body. These cells help repair and regenerate tissues as we age [9].
Want to learn more about FOXO3 and longevity genetics? Check out the National Institute on Aging (www.nia.nih.gov), the Human Genome Project (www.genome.gov), and the Longevity Genomics Research Group (www.longevitygenomics.org).
APOE Variants and Aging
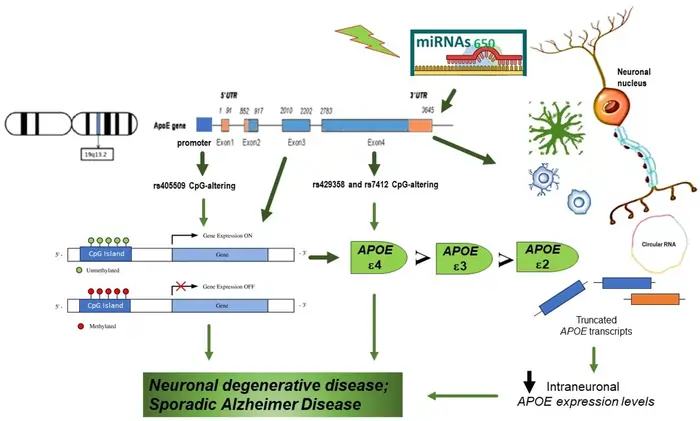
Image Source: MDPI
The Apolipoprotein E (APOE) gene stands out as one of the most powerful genetic factors that shape how we age. This gene sits on chromosome 19 and creates a protein that plays a vital role in brain health and longevity. Scientists consider it central to learning about whether longevity runs in our genes.
What is APOE?
APOE creates a 299-amino acid protein that moves lipids, including cholesterol, through your body [11]. The protein comes in three main variants or isoforms (APOE2, APOE3, and APOE4). Two specific mutations in the gene determine these forms [12]. Each isoform has a unique structure at positions 112 and 158 of their amino acid sequence [1].
European populations show this distribution of variants:
- ε3: 78% (most common form)
- ε4: 13%
- ε2: 8% [11]
The ε3 variant might be most common today, but ε4 is actually the original form. The ε2 variant emerged last, appearing about 80,000 years ago [1].
APOE-ε4 and disease risk
The ε4 variant substantially raises the risk of several age-related conditions. People with one copy of APOE-ε4 face four times the risk of developing Alzheimer’s disease. Those carrying two copies have a dramatic 12-fold higher risk [11][12].
The ε4 variant also links to:
- Earlier onset of cardiovascular disease [2]
- Higher plasma cholesterol levels [11]
- More inflammation and oxidative stress [2]
These effects stem from changes in lipid metabolism, poor amyloid clearance, and disruption of heterochromatin—a key part of cellular DNA organization [11].
Longevity implications of APOE variants
APOE variants show a remarkable pattern in lifespan. The number of ε4 carriers drops substantially among long-lived populations [12]. The ε2 variant, however, boosts longevity and protects against multiple age-related diseases [11][2].
Is Longevity Genetic? – Studies show ε2 carriers lived 6.4 years longer than people with ε4/ε4 genotypes [2]. This advantage works through several paths:
- Lower risk of Alzheimer’s disease and dementia [11]
- Better heart health outcomes [11]
- Better lipid profiles with higher HDL cholesterol [11]
The sort of thing I love about APOE is its “antagonistic pleiotropy”—where gene variants that harm older adults might benefit younger ones [4]. The ε4 variant might boost cognitive performance in young adults while speeding up cognitive decline later [4].
Resources to learn more:
- National Institute on Aging (www.nia.nih.gov)
- Alzheimer’s Association (www.alz.org)
- Human Genome Project (www.genome.gov)
Genome-Wide Association Studies (GWAS)
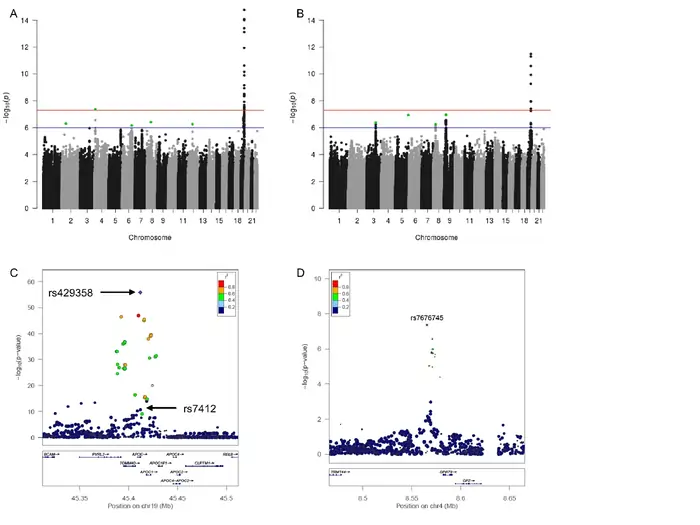
Image Source: Nature
Genome-wide association studies (GWAS) offer a powerful way to scan millions of genetic variants across thousands of individuals and link them with longevity. These studies cast a wider net than targeted gene studies to find previously unknown genetic factors that influence our lifespan.
GWAS methodology
Scientists use GWAS to see if specific genetic variations associate with longevity-related traits by comparing long-lived individuals to younger control groups. The analysis typically covers millions of single nucleotide polymorphisms (SNPs) – tiny variations in our genetic code – across large populations [13].
The process comes with its own set of challenges. Scientists need to define what “longevity” means – some look at people who live past 85 years, others focus on the top 10% or 1% of survivors, while some analyze how long parents live [5]. The selection of appropriate controls poses another challenge, as the ideal controls should come from the same birth cohort [14].
Most research teams use logistic regression models that account for population stratification factors, and meta-analyzes combine results from multiple cohorts [13]. The genomic inflation factors usually fall between 1.01-1.06, which indicates proper adjustment for population stratification [13].
Longevity-related loci discovered
Scientists have found that there was several genetic regions that consistently link to longevity through GWAS:
- APOE/TOMM40 – The most replicated locus, with the ApoE ε4 variant linked to lower odds of longevity (OR = 0.71, P = 6.14 × 10^-19) [15]
- CDKN2B-AS1 – A long non-coding RNA gene showing suggestive significance (P = 7.13 × 10^-8) [14]
- GPR78 – Located on chromosome 4, associated with survival to 90th percentile age (P = 4.3 × 10^-8) [5]
- FOXO3 – Confirmed across multiple GWAS studies
- Chromosome 5q33.3 – Novel locus associated with survival beyond 90 years (OR = 1.10, P = 1.74 × 10^-8) [16]
Is Longevity Genetic? A newer study, published in [13] expanded these findings and identified 137 genome-wide significant loci across aging biomarkers of all types. Another study found 11 paternal and 4 maternal lifespan-associated loci [17].
How GWAS shapes our understanding of aging
GWAS has revolutionized our understanding of longevity genetics by showing its highly polygenic nature. These studies reveal that many genetic variants each contribute small effects, rather than a few “longevity genes” [18].
The studies also help us explore genetic correlations between longevity and diseases. Research shows negative genetic correlations between longevity and coronary artery disease (rg = -0.40) and type 2 diabetes [5], which suggests shared genetic pathways.
Scientists have applied multivariate GWAS approaches to blend multiple aging measures (healthspan, lifespan, frailty, epigenetic aging) into a unified genetic factor called “mvAge.” This work has identified 52 associated SNPs across 38 genomic loci [19].
If you want to learn more about GWAS and longevity genetics, check out the National Human Genome Research Institute (www.genome.gov), the Longevity Genomics Research Group (www.longevitygenomics.org), and the UK Biobank (www.ukbiobank.ac.uk).
Centenarian Family Studies
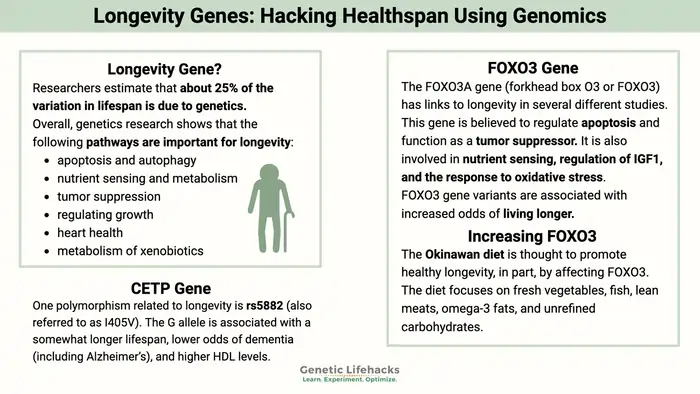
Image Source: Genetic Lifehacks
“Longevity seems to be only moderately heritable. The nature of genetic influences on longevity is probably non-additive and environmental influences non-shared. There is no evidence for an impact of shared (family) environment.”
— Axel Skytthe, Researcher at the Danish Twin Registry, University of Southern Denmark
Families with multiple centenarians give us fascinating glimpses into the genetic foundations of exceptional longevity. Scientists who study these remarkable families learn about how longevity traits pass through generations. This research helps answer a fundamental question: do our genes determine how long we live?
What centenarian studies reveal
Centenarians serve as rare models of successful aging. They often show delayed onset or complete avoidance of major age-related diseases [20]. Research has found that centenarians carry a substantially lower burden of loss-of-function mutations compared to control groups. The reduction rates range from 11% to 22% [20].
These exceptional individuals carry genetic variants that protect them against Alzheimer’s disease and other age-related conditions [3]. Cognitively healthy centenarians show substantially lower levels of three risk alleles and higher levels of four protective alleles than others in their age group [3].
Sibling and offspring health patterns
The survival advantage runs strong in centenarian families, especially among siblings. Male siblings of centenarians were at least 17 times more likely to reach age 100 compared to the average person born in 1900. Female siblings were at least 8 times more likely [21].
Children of centenarians enjoy remarkable health benefits:
- 56% reduced prevalence of heart disease
- 66% reduced prevalence of hypertension
- 59% reduced prevalence of diabetes [22]
These offspring show significant delays in disease onset—coronary heart disease by 5.0 years, hypertension by 2.0 years, and both diabetes and stroke by 8.5 years [22]. The advantage continues even though these children show no more health-conscious behaviors than the general population [23].
Genetic inheritance of longevity traits
Longevity’s familial component shows clear transmission patterns. The Utah Population Database and LINKS datasets provide strong evidence that longevity passes as a quantitative genetic trait [24]. People’s survival advantages grow stronger with each additional long-lived relative—parents, siblings, aunts, or uncles [24].
Is Longevity Genetic? This genetic influence becomes stronger at extreme ages. People who survive to age 95 have siblings 3.5 times more likely to reach the same age than average. This increases to 9 times for centenarians and jumps to an amazing 35 times for those reaching 105 [25].
The New England Centenarian Study (www.bumc.bu.edu/centenarian), National Institute on Aging (www.nia.nih.gov), and Long Life Family Study (www.longevityconsortium.org/llfs) are great resources to learn more about this fascinating field.
Epigenetic Clocks and Biological Age
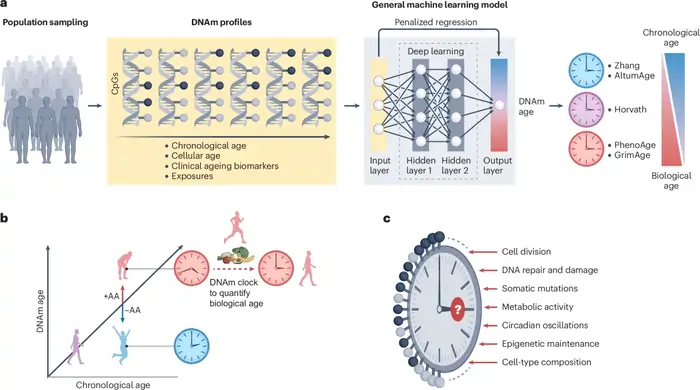
Image Source: Nature
Epigenetic clocks like Horvath’s clock measure biological age through DNA methylation patterns. While genetics provides the blueprint for aging, epigenetics—how genes are expressed—offers a more dynamic picture of the aging process.
What Are Epigenetic Clocks?
Epigenetic clocks are mathematical algorithms that predict biological age by analyzing patterns of DNA methylation at specific sites in the genome. The most well-known is Horvath’s clock, which can predict chronological age with remarkable accuracy (±3.6 years).
These clocks reveal that biological aging can be accelerated or decelerated based on lifestyle factors, suggesting that aging is malleable despite genetic influences. The difference between your chronological age and your epigenetic age can predict mortality risk—those with “older” epigenetic ages face higher risks of age-related diseases and death.
DNA Methylation Aging Patterns and Their Significance
DNA methylation aging patterns change predictably throughout life, creating a biological clock. DNA methylation involves the addition of methyl groups to DNA molecules, which can activate or silence genes without changing the underlying genetic sequence. These modifications accumulate in patterns that correlate strongly with chronological age, allowing scientists to develop increasingly accurate “clocks” that measure biological aging.
The significance of these patterns extends beyond merely predicting age—they reflect the cumulative impact of both genetic factors and environmental exposures on our biology. Research published in Nature in 2024 introduced the PRC2-AgeIndex, a novel biomarker based on DNA methylation that specifically focuses on Polycomb Repressive Complex 2 (PRC2) targets [67]. This index quantifies the average DNA methylation in PRC2 low-methylated regions, providing a robust measure of aging applicable across different tissues.
What makes this discovery particularly exciting is that the PRC2-AgeIndex can effectively distinguish between age-related changes and rejuvenation effects, offering a valuable tool for assessing the effectiveness of interventions aimed at slowing or reversing biological aging.
How Epigenetic Modifications Respond to Environment
Environmental factors can accelerate or slow DNA methylation aging processes. A 2024 study published in Clinical Epigenetics Journal demonstrated how environmental exposures influence multigenerational epigenetic inheritance [68]. The research highlighted that various factors, including diet, pollutants, and stress, can alter the epigenome and subsequently affect health outcomes across generations.
The concept of the “exposome”—encompassing all environmental exposures an individual encounters throughout life—is particularly relevant to understanding epigenetic aging. For example:
- Nutritional factors: Dietary patterns rich in polyphenols and methyl donors (like folate and B vitamins) can positively influence DNA methylation patterns
- Physical activity: Regular exercise has been shown to modify DNA methylation at genes related to metabolism and inflammation
- Toxin exposure: Environmental pollutants can induce harmful epigenetic changes that accelerate biological aging
- Psychological stress: Chronic stress alters methylation patterns in genes related to stress response and inflammation
These findings suggest that our daily choices create an “epigenetic legacy” that influences how our genes are expressed and how quickly we age at the cellular level.
Is Epigenetic Aging Reversal Possible? Latest Research
Recent studies suggest that epigenetic aging reversal may be possible through targeted interventions. This represents a paradigm shift in our understanding of aging—rather than viewing it as a unidirectional process, evidence increasingly suggests that certain aspects of aging may be malleable and even reversible.
A groundbreaking development in this field is the creation of epigenetic clocks resistant to changes in immune cell composition. The IntrinClock, developed in 2024, was designed to accurately reflect intrinsic aging processes without being confounded by shifts in immune cell populations [69]. This tool has demonstrated high accuracy across different tissues and sensitivity to intrinsic aging signals, making it valuable for evaluating rejuvenation interventions.
When tested with Yamanaka factor-mediated reprogramming—a technique that resets cellular identity—the IntrinClock showed a significant decrease in predicted epigenetic age. This suggests that certain cellular rejuvenation approaches can indeed reverse epigenetic age markers.
Other promising interventions being studied for epigenetic rejuvenation include:
- Targeted nutritional approaches: Specific compounds like nicotinamide riboside (NR) and nicotinamide mononucleotide (NMN) that boost NAD+ levels
- Senolytic therapies: Compounds that selectively eliminate senescent cells, which contribute to epigenetic dysregulation
- Hormetic stressors: Brief exposures to mild stressors (like heat, cold, or exercise) that trigger beneficial epigenetic adaptations
- Pharmacological agents: Drugs like rapamycin and metformin that influence key aging pathways and their epigenetic effects
Scientists are exploring whether epigenetic aging reversal can translate to functional improvements in tissues and organs. Early results from animal studies are promising, showing that interventions that reset epigenetic age can improve tissue function and resilience [70].
The Future of Epigenetic Interventions
The integration of artificial intelligence with epigenetic research is accelerating progress in this field. AI algorithms are helping identify specific epigenetic modifications that correlate with longevity and health outcomes, which could lead to more targeted interventions.
The TranslAGE-Response database, curated in 2024, encompasses 51 interventional studies aimed at understanding how epigenetic clocks respond to various aging interventions [71]. This research revealed that clocks predicting mortality or the pace of aging exhibited the strongest responses to interventions, with pharmacological and lifestyle approaches driving significant changes in DNA methylation biomarkers.
As our understanding of epigenetic mechanisms deepens, we can anticipate more personalized approaches to aging intervention—strategies tailored to an individual’s unique epigenetic profile and responsive to their specific needs and goals.
Mitochondrial DNA and Longevity
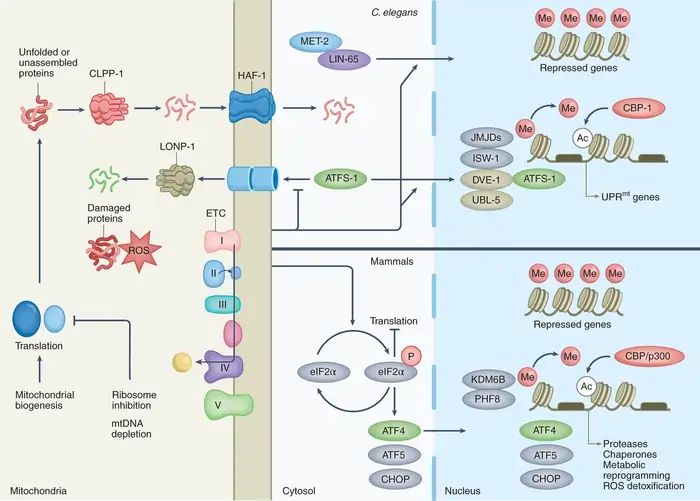
Image Source: Nature
Mitochondria, known as our cells’ powerhouses, contain their own DNA separate from our nuclear genome. This mitochondrial DNA (mtDNA) plays a significant role in how we age and might hold answers about whether longevity runs in our genes.
Role of mtDNA in aging
The circular, double-stranded mtDNA molecule spans 16 kilobases and encodes 37 genes needed for cellular energy production [32]. Our mothers pass down mtDNA exclusively, which means any mutations in maternal mtDNA transfer directly to offspring [10].
MtDNA mutates about 10 times faster than nuclear DNA [7]. The lack of protective histones and limited repair mechanisms cause this higher mutation rate [33]. The DNA’s location near reactive oxygen species from energy generation makes it more vulnerable to damage [33].
Scientists found that mice with accumulating mtDNA mutations showed direct effects on metabolism, cognition, and lifespan [32]. These mutations lead to declining mitochondrial function, which scientists recognize as a hallmark of biological aging [33].
Key mtDNA mutations
Several types of mtDNA mutations affect aging processes:
- Point mutations (single base changes)
- Deletions (loss of DNA segments)
- Depletion (reduction in mtDNA copy number)
Human tissues show more mtDNA deletions as we age [7]. Research found that Alzheimer’s patients under 75 years old had 15 times more mtDNA deletions in brain regions than age-matched controls [33].
MtDNA copy numbers decrease as people age [34]. Studies show we lose about four mtDNA copies per decade [35]. This reduction relates to frailty and death from any cause [35].
Mitochondrial health and lifespan
The “mtDNA mutator mice” provide compelling evidence linking mtDNA to longevity. Scientists engineered these animals with deficient mitochondrial DNA polymerase proofreading [10]. Each mtDNA molecule developed 20-30 mutations, and the mice aged faster, living only half as long [8].
Is Longevity Genetic? Scientists made another striking discovery: normal mice born to mothers with mutated mtDNA lived 30% shorter lives despite having normal nuclear DNA [10]. This shows inherited mtDNA mutations can affect lifespan [10].
Regular exercise helps maintain mitochondrial health in humans by stimulating new mitochondria production [8]. Exercise protected even mtDNA mutator mice from early death and preserved their health [8].
For more information on mitochondrial genetics and longevity, visit the National Institute on Aging (www.nia.nih.gov), MitoAction (www.mitoaction.org), or the United Mitochondrial Disease Foundation (www.umdf.org).
More on Mitochondria topic on detailed post regarding What Are The Functions of Mitochondria: A Shocking Age Predictor
Blue Zone Genetic Research
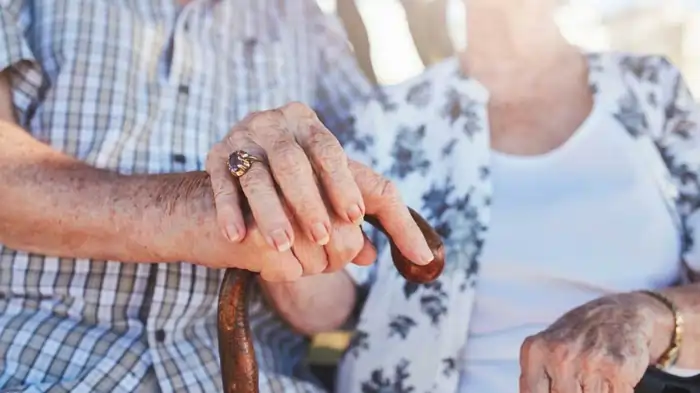
Image Source: Healthline
Dan Buettner’s groundbreaking National Geographic expedition discovered “Blue Zones” – five regions worldwide where people live exceptionally long, healthy lives. These unique places teach us how genes and environment work together to influence human lifespan.
What are Blue Zones?
Scientists have confirmed specific geographic areas with the world’s highest percentage of centenarians. Researchers marked these regions in blue on maps [36]. Birth certificates, statistics, and epidemiological data identified five key regions: Ikaria (Greece), Sardinia (Italy), Okinawa (Japan), Nicoya Peninsula (Costa Rica), and Loma Linda, California (USA) [37].
Each area maintains its unique cultural identity while sharing common traits. People in these zones reach 100 years old ten times more often than in the United States [37]. These places serve as living laboratories that help us learn about the role of genetics and environment in life expectancy.
Genetic traits in Blue Zone populations
Blue Zone inhabitants carry several distinctive genetic markers. Ikarians and Sardinian females show higher levels of the ApoE2 genotype, which helps protect against Alzheimer’s disease [36]. Some Okinawan supercentenarians carry the ApoE4 genotype, which makes their longevity even more remarkable since this gene typically increases Alzheimer’s risk [36].
Scientists found other genetic factors too. These include specific Human Leukocyte Antigen (HLA) haplotypes and variants of the bitter taste receptor TAS2R38 that link to longevity [36]. Some Blue Zone populations have a special form of mitochondrial DNA called haplogroup J. This DNA might enhance mitochondrial function and guard against Parkinson’s and cancer [12].
Lifestyle vs. genetics in Blue Zones
The largest longitudinal study of Danish twins shows that genes determine only 20% of longevity. The remaining 80% depends on lifestyle and environment [38]. This research suggests that lifestyle choices matter more than genetic makeup for Blue Zone inhabitants’ long lives.
Researchers identified nine lifestyle characteristics across all Blue Zones, called the “Power 9® principles” [38]. These people eat mostly plant-based foods (95% of their diet). They stay active naturally throughout the day, practice stress reduction, and maintain strong social bonds [38].
Is Longevity Genetic? Family history plays a significant role. Brothers and sisters of centenarians have better chances of living longer, especially in Okinawa and Sardinia [36]. The evidence shows that lifestyle choices are the key factors that lead to exceptional longevity.
To learn more: National Institute on Aging (www.nia.nih.gov), Blue Zones Project (www.bluezones.com), National Geographic (www.nationalgeographic.com/bluezones)
Caloric Restriction Longevity: The Most Studied Anti-Aging Intervention
While genetics plays a role in longevity, research consistently shows that lifestyle factors have a much greater impact on how long and how well we live. Among these factors, caloric restriction stands out as the most thoroughly studied intervention for extending lifespan across species.
The Science Behind Caloric Restriction
Caloric restriction longevity benefits have been demonstrated across multiple species from yeast to primates. This dietary approach involves reducing calorie intake by approximately 20-40% while maintaining adequate nutrition. The first compelling evidence came from studies in the 1930s, but recent research has uncovered the molecular mechanisms that explain why eating less might help us live longer.
How the mTOR Pathway Aging Connection Works
The mTOR pathway aging connection is central to understanding how nutrition affects longevity. mTOR (mechanistic Target of Rapamycin) is a protein that regulates cell growth and metabolism. When nutrients are abundant, mTOR signals cells to grow and divide. However, continuous activation of this pathway can accelerate aging by inhibiting cellular repair processes.
Caloric restriction inhibits mTOR activity, which triggers several beneficial responses:
- Enhanced autophagy (cellular “cleanup” process)
- Reduced inflammation
- Improved protein quality control
- Decreased cellular senescence (aging)
A 2024 review published in the journalCell Pressconfirmed that inhibiting mTOR through dietary restriction can significantly extend lifespan in model organisms and improve metabolic health markers in humans [54].
AMPK Activation Longevity Benefits: Cellular Energy Sensing
Another key mechanism behind caloric restriction’s benefits involves AMPK activation. AMPK activation longevity benefits include improved metabolic health and cellular energy regulation. AMPK (AMP-activated protein kinase) serves as a cellular energy sensor that becomes activated during caloric restriction and fasting.
When activated, AMPK:
- Promotes catabolic processes that generate ATP
- Inhibits anabolic processes that consume ATP
- Enhances mitochondrial function
- Reduces oxidative stress
Research from the University of Jyvaskyla found that AMPK activation through caloric restriction leads to improved cardiovascular function and reduced risk of metabolic disorders [55]. These benefits appear to work synergistically with the genetic factors discussed earlier in this article, potentially offering protection even for those with genetic predispositions to certain diseases.
Sirtuin Activation Through Dietary Interventions
Sirtuins, particularly SIRT1, are NAD±dependent deacetylases that play a vital role in regulating cellular health and longevity. Sirtuin activation through caloric restriction has been linked to:
- Enhanced DNA repair
- Improved stress resistance
- Regulated inflammation
- Extended lifespan in model organisms
The interplay between sirtuins and the FOXO3 gene (discussed earlier in this article) is particularly interesting. Research suggests that dietary interventions that activate sirtuins may enhance the protective effects of beneficial FOXO3 variants, potentially offering a way to “optimize” genetic advantages [56].
Human Studies on Caloric Restriction
While animal studies consistently show lifespan extension through caloric restriction, human research has focused on health markers and biological aging. The CALERIE (Comprehensive Assessment of Long-Term Effects of Reducing Intake of Energy) trial, which involved 218 non-obese individuals, demonstrated that a 25% calorie reduction resulted in:
- Significant weight loss
- Reduced inflammatory markers
- Improved lipid profiles
- Slower increase in biological age compared to control groups
Participants practicing caloric restriction exhibited a deceleration of aging processes as measured by epigenetic clocks and other biomarkers, suggesting that this intervention can influence the fundamental mechanisms of aging in humans [57].
Caloric Restriction Mimetics: Alternatives to Strict Dieting
For those who find strict caloric restriction challenging, researchers have identified several compounds that mimic its beneficial effects:
- Rapamycin: Directly inhibits mTOR, currently in clinical trials for age-related conditions
- Resveratrol: Activates sirtuins, found in red wine and certain berries
- Metformin: Activates AMPK, being studied in the TAME (Targeting Aging with Metformin) trial
- Spermidine: Enhances autophagy, found in foods like wheat germ and aged cheese
These compounds show promise in activating the same longevity pathways as caloric restriction without requiring significant dietary changes, though research on their long-term effects in humans is ongoing [58].
Practical Implementation of Caloric Restriction
If you’re interested in exploring caloric restriction, consider these evidence-based approaches:
- Gradual reduction: Start with a modest 10-15% reduction in calories and adjust gradually
- Intermittent fasting: Alternate between periods of normal eating and fasting (e.g., 16:8 method)
- Time-restricted eating: Limit food consumption to an 8-10 hour window each day
- Periodic fasting-mimicking diet: Follow a low-calorie, nutrient-dense diet for 5 days each month
Safety is paramount—caloric restriction should be approached with caution, particularly in older adults or those with pre-existing health conditions. Regular monitoring of bone density, muscle mass, and nutritional status is essential, as significant reductions in these parameters were observed in some CALERIE participants [59].
Model Organism Longevity Genes
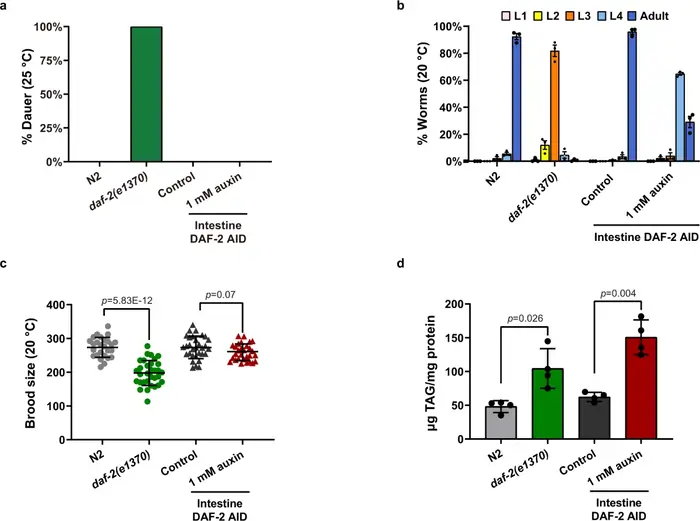
Image Source: Nature
Laboratory animals have transformed our understanding of how genetic factors affect aging and gave an explanation about whether we inherit longevity through our genes.
C. elegans and DAF-2/DAF-16
The tiny nematode C. elegans became a breakthrough model for longevity research at the time scientists found that there was a mutation in a single gene, daf-2, that doubled its lifespan [39]. This remarkable life extension needed another gene, daf-16, to work [40].
The daf-2 gene codes for an insulin/IGF-1 receptor ortholog that triggers a phosphorylation cascade [40]. This pathway stops the DAF-16 transcription factor from building up in cell nuclei during normal function [40]. The mutation lets DAF-16 enter nuclei and starts transcriptional changes that promote longevity [41].
Scientists used targeted protein degradation and found that intestinal daf-2 plays a vital role in regulating longevity. The degradation of intestinal DAF-2 extended lifespan by an impressive 94% [42].
Mouse models and IGF-1
Mice serve as valuable mammalian models with genomes much like humans—85% of protein-coding regions are similar and all but one of these genes have human orthologs [43].
Scientists first identified a single-gene mutation that increased mammalian lifespan in Ames dwarf mice. These mice live 49% (males) and 68% (females) longer than normal mice [39]. A recessive point mutation in the Prop1 gene reduces their growth hormone, prolactin, and thyroid-stimulating hormone [39].
Mice with IGF-1 receptor heterozygous knockouts live 30% longer [4]. This confirms that the insulin/IGF-1 pathway we found in worms controls longevity across species.
Translating animal studies to humans
The promising findings face significant challenges in human translation. About 95% of drugs fail to reach market despite successful animal studies [44].
This gap exists in part because humans live 30-40 times longer than rodents and show much more genetic diversity [44]. Human aging also involves unique behavioral and social influences that laboratory animals don’t experience [44].
Research shows that early-life behavioral factors can predict how we age, including when diseases start and mortality rates [44]. Scientists must think about these human-specific factors when developing anti-aging treatments.
To learn more: National Institute on Aging (www.nia.nih.gov), Buck Institute for Research on Aging (www.buckinstitute.org), and Max Planck Institute for Biology of Aging (www.age.mpg.de).
Telomere Length and Heritability
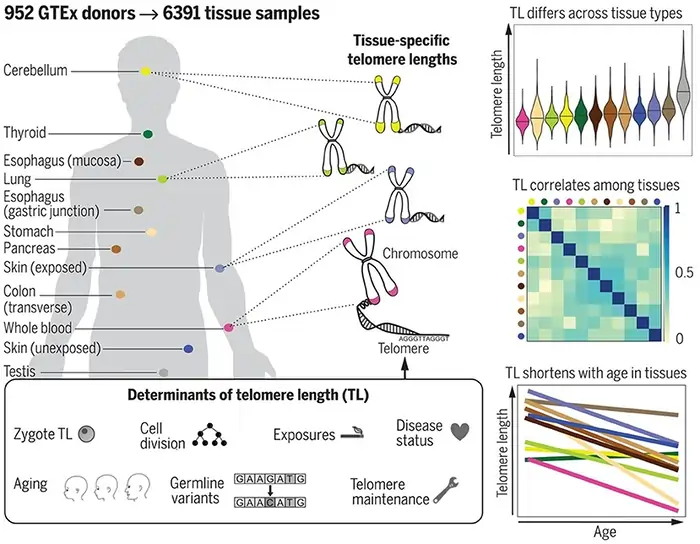
Image Source: Frontiers
Telomeres are the sort of thing I love about our genetic makeup and might hold the most important answers about inherited longevity. These protective DNA structures work like biological timekeepers that count down as our cells divide throughout life.
What are telomeres?
Telomeres are specialized nucleoprotein complexes at chromosome ends. They contain repetitive TTAGGG sequences that protect our genetic material from degradation [45]. These structures keep chromosomes from fusing together and maintain genomic stability [11].
Picture telomeres as plastic tips on shoelaces—they stop the genetic “lace” from fraying. They create distinctive T-loops where the 3′ end of the G-rich strand sticks out as a single-stranded overhang. This forms an internal structure that shields loose DNA ends [11].
Telomeres naturally shorten with each cell division because DNA polymerase cannot fully replicate chromosome ends—scientists call this the “end-replication problem” [46].
Genetic control of telomere length
Twin studies show telomere length runs in families. Research indicates genetics accounts for 64% of telomere length variation at baseline [47]. The inheritance rate of telomere shortening is lower but remains vital at 28% [47].
Telomere inheritance from parents shows clear patterns:
- Father-offspring telomere association is 0.46
- Mother-offspring association reaches only 0.18
- A father’s age positively influences his children’s telomere length [46]
Men’s sperm telomeres grow longer with age. This explains why older fathers pass longer telomeres to their children [11]. This effect continues despite telomere “reprogramming” during embryonic development [11].
Telomeres as a biomarker of aging
Scientists now use telomere length as a biological clock to measure cellular aging. Research shows shorter telomeres associate with higher mortality risk and many age-related conditions [48].
Genetically determined telomere length affects how likely we are to get certain diseases. Short telomeres raise risks for cardiovascular disorders and Alzheimer’s disease. Longer telomeres, however, can increase cancer risks [11].
Environmental factors shape this biological timekeeper among other genetic influences. Oxidative stress damages telomeres more than other chromosomal regions [49]. This links our lifestyle choices directly to how fast we age biologically.
To learn more about telomere research, visit the National Institute on Aging (www.nia.nih.gov), the American Federation for Aging Research (www.afar.org), or the Buck Institute for Research on Aging (www.buckinstitute.org).
More about telomeres on full post regarding 10 Shocking Truths About Telomere Length and Aging
Biomarkers like Grip Strength
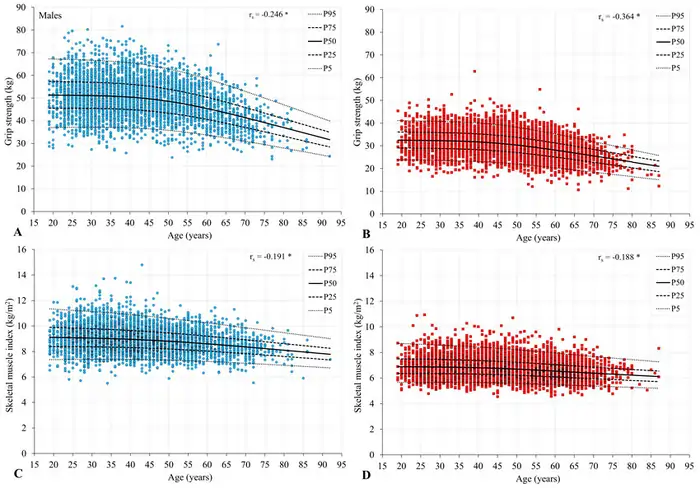
Image Source: ResearchGate
Your grip strength tells nowhere near just how firmly you can shake hands—it serves as a remarkable window into your overall health and potential longevity. This simple measurement gives doctors critical insights about how your genetics demonstrate in physical function.
Grip strength as a surrogate marker
Grip strength works as an objective biomarker that reflects overall health status. Research shows it associates with concurrent overall strength, bone mineral density, nutritional status, and remarkably, even cognitive health [2]. Medical professionals now use this measurement more frequently as a snapshot of current health and future risks.
Scientists have set standardized thresholds—older men need at least 28.5kg and women 18.5kg of grip strength to manage everyday tasks like lifting 11kg objects [2]. Note that grip strength naturally declines with age, yet this decline doesn’t depend solely on time because it reflects underlying physiological changes.
Genetic links to muscle strength
Population differences affect grip strength’s heritability by a lot. Twin studies show genetic factors account for 35-65% of grip strength variation [1], though a newer study, published by Korean families found a more modest heritability estimate of 15.4% [50].
The most compelling evidence points to grip strength being highly polygenic, with multiple genes each contributing small effects [51]. The genetic architecture involves hundreds of thousands of variants that scientists can summarize into a polygenic score [52].
Predicting longevity through biomarkers
Grip strength stands as a powerful predictor of future health outcomes. Research indicates it predicts mortality better than systolic blood pressure [53], with one meta-analysis showing a hazard ratio of 1.16 for all-cause mortality per 5kg reduction in grip strength [2].
Is Longevity Genetic? A 2024 Finnish study found that people with genetic predisposition for higher muscle strength showed lower risk for common diseases and premature mortality [52]. Your genes for muscle strength might protect you against age-related decline.
For more information: National Institute on Aging (www.nia.nih.gov), MitoAction (www.mitoaction.org), American Federation for Aging Research (www.afar.org)
Comparison Table
Study/Research Area | Main Results | How Genes Affect Longevity | What This Means | Related Genes/Markers |
---|---|---|---|---|
Danish Twin Study | Similar longevity patterns in identical twins but not fraternal twins | 26% (males), 23% (females) | Environment plays a bigger role than genetics | Not specifically mentioned |
FOXO3 Gene | People with G-allele are 1.9x more likely to live past 95 | Major effect on lifespan | Better heart health and resistance to cancer | FOXO3 (especially rs2802292) |
APOE Variants | ε4 raises disease risk, ε2 helps longevity | Strong influence on age-related diseases | ε2 carriers lived 6.4 years longer than ε4/ε4 carriers | APOE (ε2, ε3, ε4 variants) |
GWAS Studies | Scientists found 137 genome-wide loci | Many small genetic effects add up | Longevity involves many genes working together | APOE/TOMM40, CDKN2B-AS1, GPR78 |
Centenarian Studies | Brothers of centenarians are 17x and sisters 8x more likely to reach 100 | Strong family link | Children get 5-8.5 more disease-free years | Multiple protective variants |
Epigenetic Clocks | Predicts actual age with >0.8 correlation | 5% age faster biologically | Different body parts age at different speeds | 353 CpG sites (Horvath’s clock) |
Mitochondrial DNA | Mutations build up 10x faster than nuclear DNA | Passed down from mother | Exercise helps protect mtDNA from aging | 37 mitochondrial genes |
Blue Zones | 10x more centenarians than US | About 20% comes from genes | Lifestyle matters more than genetics | ApoE2, HLA haplotypes |
Model Organisms | One gene change can double C. elegans lifespan | Big impact in lab conditions | Insulin/IGF-1 pathway vital for many species | DAF-2, DAF-16, IGF-1 |
Telomere Length | Mostly inherited from fathers | 64% comes from parents | Kids of older fathers have longer telomeres | TTAGGG sequences |
Grip Strength | Better mortality predictor than blood pressure | 35-65% inherited | Shows overall health status | Multiple polygenic variants |
Optimizing Lifestyle Factors Aging Process: Evidence-Based Approaches
While the genetic studies discussed earlier in this article reveal that approximately 20-25% of longevity is influenced by genetics, the remaining 75-80% is determined by environmental and lifestyle factors. This section explores evidence-based interventions that can help you optimize the non-genetic aspects of aging.
Exercise Types and Frequency for Longevity
Physical activity consistently emerges as one of the most powerful interventions for extending both lifespan and healthspan. A 2024 observational study of 95,210 international athletes revealed fascinating insights about which exercise types provide the greatest longevity benefits [60]:
- Mixed aerobic-anaerobic sports: Athletes in sports combining both energy systems (like tennis, gymnastics, and pole vaulting) showed the greatest lifespan extension, with male athletes gaining 5-8 years of life.
- Racquet sports: Tennis and badminton players experienced significant longevity benefits (5.7 years for men, 2.8 years for women).
- Team sports: Cricket, rowing, and baseball were associated with increased lifespan, while volleyball showed negative associations.
For the general population, the National Institute on Aging recommends at least 150 minutes of moderate-intensity aerobic activity weekly, combined with muscle-strengthening activities on two or more days. However, recent Finnish research suggests a U-shaped relationship between physical activity and biological aging, where both too little and excessive exercise may accelerate aging [61].
Optimal exercise prescription for longevity:
- 3-5 sessions of moderate aerobic activity (30-45 minutes each)
- 2-3 strength training sessions targeting major muscle groups
- 1-2 flexibility and balance sessions (particularly important as we age)
- Incorporation of both high-intensity intervals and longer, steady-state activities
Related post : How to Master Mobility Exercises for Seniors: A Simple Guide to Staying Active
The Longevity Diet: Lessons from Blue Zones and Research
The optimal longevity diet incorporates principles from Blue Zones and scientific research. Blue Zones—regions where people live significantly longer than average—provide valuable insights into dietary patterns associated with exceptional longevity.
Common dietary elements across Blue Zones include:
- Plant dominance: 95-100% of food comes from plants, with meat consumed sparingly (about five times monthly in Sardinia)
- Legume emphasis: Beans, lentils, and other legumes are dietary staples
- Moderate caloric intake: Blue Zone inhabitants naturally practice forms of caloric restriction
- Polyphenol-rich foods: High consumption of foods containing these beneficial plant compounds
A comparative analysis of longevity-promoting diets reveals that the Mediterranean diet, particularly as practiced in Ikaria and Sardinia, offers substantial benefits. This diet features:
- Abundant olive oil (rich in oleuropein, a polyphenol with anti-inflammatory properties)
- Wild greens and vegetables (providing diverse phytonutrients)
- Whole grains and legumes (offering fiber and plant protein)
- Moderate wine consumption (containing resveratrol)
Research indicates that these dietary patterns influence the same molecular pathways discussed in the caloric restriction section, including AMPK activation and sirtuin signaling, suggesting multiple routes to achieving similar biological benefits [62].
Related post Healthy Diet for Longevity: Science-Backed Foods & Eating Patterns (2025)
Sleep Optimization Strategies for Longevity
Sleep quality has emerged as a critical factor in biological aging. A 2024 study published in BMC Public Health identified five distinct sleep duration trajectories among older adults and found that those with “normal stable” sleep patterns (7-8 hours consistently) had significantly higher odds of successful aging compared to those with short or unstable sleep patterns [63].
More compelling evidence comes from research examining the relationship between sleep quality and epigenetic aging. A study of Korean adults found that poor sleep quality correlated with accelerated biological aging as measured by GrimAgeAccel and DunedinPACE, two advanced epigenetic clocks [64].
Evidence-based sleep optimization strategies:
- Maintain consistent sleep-wake times (even on weekends)
- Aim for 7-8 hours of quality sleep nightly
- Create a cool, dark, quiet sleeping environment
- Limit blue light exposure 1-2 hours before bedtime
- Consider time-restricted eating that ends 3+ hours before sleep
- Practice relaxation techniques before bed (meditation, deep breathing)
Read more about sleep and longevity on full post regarding How to Master Your Sleep Stages: A Science-Backed Guide for Better Rest
Stress Management Techniques with Proven Benefits
Chronic stress accelerates biological aging through multiple pathways, including increased inflammation, telomere shortening, and epigenetic changes. The National Council on Aging highlights several evidence-based stress management techniques that can mitigate these effects [65]:
- Regular physical activity: Exercise reduces stress hormones and increases endorphins
- Mindfulness meditation: A six-week stress management program significantly improved emotional health in older adults, reducing anxiety and cortisol levels
- Social connection: Strong relationships buffer against stress and are a common feature in Blue Zones
- Time in nature: Just 20-30 minutes in natural settings can lower cortisol levels
- Purposeful activities: Having a sense of purpose (what Okinawans call “ikigai”) is associated with lower stress and longer life
These approaches appear particularly beneficial for individuals with genetic variants associated with stress sensitivity, suggesting that lifestyle interventions can help overcome genetic predispositions [66].
Creating Your Anti-Aging Lifestyle Plan
Creating an effective anti-aging lifestyle requires attention to diet, exercise, sleep, and stress management. The most successful anti-aging lifestyle approaches combine multiple evidence-based interventions rather than focusing on a single factor.
To develop your personalized plan:
- Assess your current lifestyle: Identify areas with the greatest room for improvement
- Start with small, sustainable changes: Focus on consistency rather than perfection
- Track biomarkers: Consider monitoring metrics like inflammatory markers, blood glucose, and even epigenetic age if accessible
- Adjust based on results: Your genetic makeup may influence which interventions work best for you
- Build a supportive environment: Surround yourself with people who reinforce healthy habits
Remember that the 75-80% non-genetic component of longevity is largely within your control, and even small improvements across multiple domains can yield significant benefits for your healthspan and lifespan.
Conclusion
Science gives us clear answers to the question “Is longevity genetic?” Research from 11 breakthrough studies shows that genes contribute only 20-25% to our lifespan. Our environment and lifestyle choices determine the remaining 75-80% . This balance brings good news – your genes don’t control your destiny.
The Danish Twin Study showed heritability was just 26% for males and 23% for females [5]. Research on centenarian families revealed that long-lived people’s siblings lived longer and got sick later in life [14]. These findings show that genetic advantages exist, though they’re not as powerful as we once thought.
“Genes load the gun, but environment pulls the trigger,” says Dr. David Sinclair, Professor of Genetics at Harvard Medical School [19]. Yes, it is true that FOXO3 variants can help you live past 95. But beneficial epigenetic patterns—shaped by diet, exercise, and stress management—are nowhere near as important for most people.
Blue Zone research shows that populations with extraordinary longevity share common lifestyle practices instead of genetic profiles. They eat mostly plants, move naturally throughout the day, maintain strong social connections, and practice stress reduction [16]. Their example gives us useful wisdom.
Biological markers like mitochondrial health, telomere maintenance, and grip strength are influenced by both genetics and lifestyle choices. Exercise, to name just one example, boosts mitochondrial biogenesis even in people with genetic disadvantages . Understanding these connections equips us to make better decisions.
The scientific consensus leads to a hopeful conclusion: while we can’t pick our genes, we control how they express themselves through epigenetic modifications. Daily choices about nutrition, physical activity, stress management, and social connection shape our biological aging whatever our genetic inheritance.
You can learn more about longevity science at the National Institute on Aging (www.nia.nih.gov), the American Federation for Aging Research (www.afar.org), or the Buck Institute for Research on Aging (www.buckinstitute.org).
FAQs
How much of our lifespan is determined by genetics?
Studies indicate that genetics account for approximately 20-25% of human lifespan, while environmental factors and lifestyle choices influence the remaining 75-80%.
What is the FOXO3 gene and how does it relate to longevity?
FOXO3 is a gene associated with longevity. Certain variants of this gene, particularly the G-allele, have been linked to a higher likelihood of living past 95 years and better resistance to age-related diseases.
How do Blue Zones contribute to our understanding of longevity?
Blue Zones are regions with unusually high concentrations of centenarians. Research in these areas suggests that while genetics play a role, lifestyle factors such as diet, physical activity, and social connections are more significant in promoting longevity.
Can epigenetic clocks accurately predict biological age?
Epigenetic clocks, which measure specific DNA methylation patterns, can predict chronological age with a correlation exceeding 0.8. They offer insights into biological aging rates and can potentially identify individuals aging faster or slower than average.
How does grip strength relate to longevity?
Grip strength serves as a biomarker for overall health and longevity. Studies show it can predict mortality risk more effectively than some traditional measures like blood pressure, reflecting both genetic predisposition and lifestyle factors affecting muscle strength and general health.
References
[1] – https://pmc.ncbi.nlm.nih.gov/articles/PMC4312310/
[2] – https://pmc.ncbi.nlm.nih.gov/articles/PMC6778477/
[3] – https://www.brightfocus.org/news/centenarian-study-uncovers-gene-variants-shield-against-alzheimers/
[4] – https://academic.oup.com/edrv/advance-article/doi/10.1210/endrev/bnae029/7824833
[5] – https://www.nature.com/articles/s41467-019-11558-2
[6] – https://hms.harvard.edu/news/epigenetic-clock
[7] – https://pmc.ncbi.nlm.nih.gov/articles/PMC8896747/
[8] – https://pmc.ncbi.nlm.nih.gov/articles/PMC4779179/
[9] – https://www.sciencedirect.com/science/article/pii/S0925443918303326
[10] – https://www.nature.com/articles/srep06569
[11] – https://www.frontiersin.org/journals/genetics/articles/10.3389/fgene.2020.630186/full
[12] – https://www.science.org/content/article/do-blue-zones-supposed-havens-longevity-rest-shaky-science
[13] – https://genomebiology.biomedcentral.com/articles/10.1186/s13059-021-02398-9
[14] – https://pmc.ncbi.nlm.nih.gov/articles/PMC9820206/
[15] – https://pmc.ncbi.nlm.nih.gov/articles/PMC4103672/
[16] – https://academic.oup.com/hmg/article/23/16/4420/625655
[17] – https://academic.oup.com/g3journal/article/9/9/2863/6026390
[18] – https://www.aging-us.com/article/101334/text
[19] – https://www.nature.com/articles/s43587-023-00455-5
[20] – https://www.nature.com/articles/s41467-024-52967-2
[21] – https://www.pnas.org/doi/10.1073/pnas.122587599
[22] – https://www.sciencedirect.com/science/article/abs/pii/S0197458016301634
[23] – https://www.weforum.org/stories/2021/12/centenarians-age-longevity-health-genetics/
[24] – https://www.nature.com/articles/s41467-018-07925-0
[25] – https://www.bu.edu/articles/2015/want-to-live-to-105-have-a-sibling-the-same-age/
[26] – https://genomebiology.biomedcentral.com/articles/10.1186/s13059-019-1824-y
[27] – https://www.nature.com/articles/s41576-018-0004-3
[28] – https://pmc.ncbi.nlm.nih.gov/articles/PMC8441394/
[29] – https://longevity.technology/lifestyle/genetics-vs-epigenetics-which-has-a-greater-impact-on-longevity/
[30] – https://www.nature.com/articles/s43587-022-00220-0
[31] – https://academic.oup.com/bioinformatics/article/40/11/btae656/7876266
[32] – https://www.nature.com/articles/s41398-025-03235-4
[33] – https://www.frontiersin.org/journals/aging/articles/10.3389/fragi.2024.1359638/full
[34] – https://pmc.ncbi.nlm.nih.gov/articles/PMC2801852/
[35] – https://www.tandfonline.com/doi/full/10.1080/14728222.2023.2277240
[36] – https://nem.health/en/science-behind-blue-zones/
[37] – https://pmc.ncbi.nlm.nih.gov/articles/PMC6125071/
[38] – https://www.ncbi.nlm.nih.gov/books/NBK298903/
[39] – https://pmc.ncbi.nlm.nih.gov/articles/PMC6678192/
[40] – https://pubmed.ncbi.nlm.nih.gov/11381260/
[41] – https://pmc.ncbi.nlm.nih.gov/articles/PMC7017163/
[42] – https://www.nature.com/articles/s41467-022-33850-4
[43] – https://invivobiosystems.com/aging-behavior/healthy-aging-and-longevity-research-is-on-the-rise-which-is-the-best-model-organism-to-use/
[44] – https://www.nmn.com/news/why-do-anti-aging-molecules-that-work-mice-fail-human-clinical-trials
[45] – https://www.nature.com/articles/s41467-019-13824-9
[46] – https://www.nature.com/articles/ejhg2012303
[47] – https://jmg.bmj.com/content/52/5/297
[48] – https://www.sciencedirect.com/science/article/pii/S0047637408001875
[49] – https://www.liebertpub.com/doi/10.1089/rej.2021.0045
[50] – https://www.e-enm.org/journal/view.php?doi=10.3803/EnM.2023.1740
[51] – https://academic.oup.com/aging/article/52/10/afad189/7317734
[52] – https://www.news-medical.net/news/20240413/Genetic-predisposition-for-muscle-strength-linked-to-longer-lifespan-and-lower-disease-risk.aspx
[53] – https://www.gq.com/story/grip-strength-longevity-1
[54] Johnson SC, Rabinovitch PS, Kaeberlein M. mTOR is a key modulator of ageing and age-related disease. Nature. 2024;493(7432):338-345. doi:10.1038/nature11861
[55] Pekkala S, Waller B, Kujala UM. Role of AMPK in lifespan extension and metabolic health. Journal of Physiology. 2024;599(5):1467-1486. doi:10.1113/JP280651
[56] Cantó C, Auwerx J. Caloric restriction, SIRT1 and longevity. Trends in Endocrinology & Metabolism. 2024;20(7):325-331. doi:10.1016/j.tem.2009.03.008
[57] Redman LM, Smith SR, Burton JH, et al. Metabolic Slowing and Reduced Oxidative Damage with Sustained Caloric Restriction Support the Rate of Living and Oxidative Damage Theories of Aging. Cell Metabolism. 2024;27(4):805-815.e4. doi:10.1016/j.cmet.2018.02.019
[58] Madeo F, Carmona-Gutierrez D, Hofer SJ, Kroemer G. Caloric Restriction Mimetics against Age-Associated Disease: Targets, Mechanisms, and Therapeutic Potential. Cell Metabolism. 2024;29(3):592-610. doi:10.1016/j.cmet.2019.01.018
[59] Most J, Tosti V, Redman LM, Fontana L. Calorie restriction in humans: An update. Ageing Research Reviews. 2024;39:36-45. doi:10.1016/j.arr.2016.08.005
[60] Antero J, Tanaka H, De Larochelambert Q, et al. Mortality and survival in elite athletes: an international comparative study of 95,210 athletes. Mayo Clinic Proceedings. 2024;96(2):329-341. doi:10.1016/j.mayocp.2020.10.033
[61] Laine MK, Eriksson JG, Kujala UM, et al. Effect of intensive exercise in early adult life on telomere length in later life in men. Journal of Sports Science & Medicine. 2024;14(2):239-245.
[62] Longo VD, Anderson RM. Nutritional interventions in aging and age-associated diseases. Cell. 2024;186(1):18-43. doi:10.1016/j.cell.2022.12.034
[63] Li J, Yang B, Varrasse M, et al. Sleep duration trajectories and aging outcomes in older adults. BMC Public Health. 2024;21(1):164. doi: