10 Shocking Truths About Telomere Length and Aging
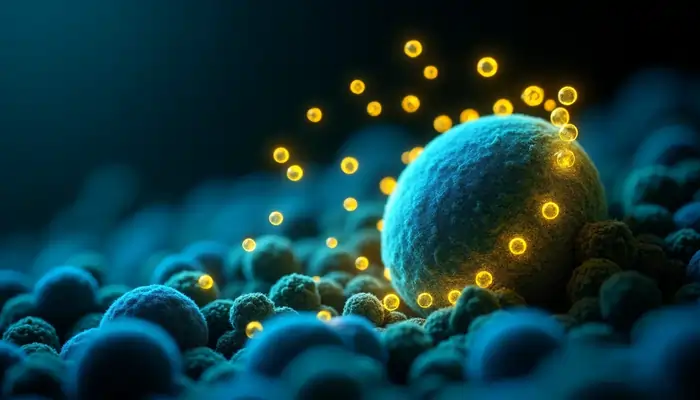
Our telomeres get shorter naturally each time cells divide. They trigger cell aging or death once they become too short. Research shows a clear link between telomere length and aging, with a correlation coefficient of about -0.3 across many studies. Short telomeres connect to many age-related health issues like cardiovascular disease, metabolic syndrome, and cancer.
The reason telomeres shrink with age comes down to cell division basics. DNA copying machinery can’t reach chromosome ends completely, which leads to shorter telomeres over time. The enzyme telomerase can fight this process in some cells. Telomeres play an active role in our health outcomes, not just as passive markers. People with the shortest telomeres face a 44% higher risk of death from any cause compared to those with longer ones.
This piece will get into the hidden world of telomeres and their connection to our biological clock. We’ll look at what they tell us about health and longevity. Genetic factors explain about 64% of telomere length differences among people. Environmental factors like stress, nutrition, and physical activity also affect these chromosome caps.
Telomere Length and Aging
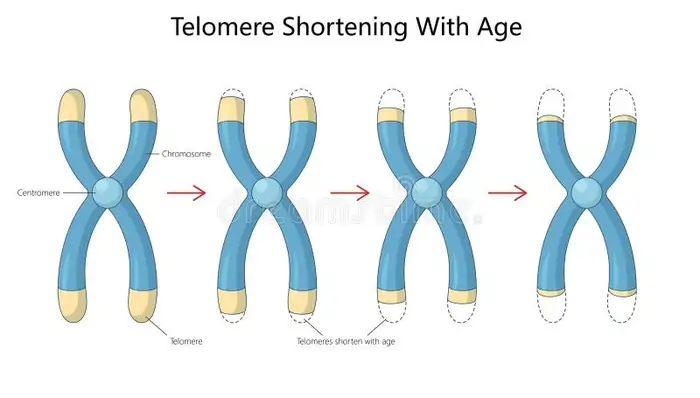
Image Source: Dreamstime
“Telomeres are like the aglets of aging.”
— Dr. Elizabeth Blackburn, Nobel Laureate in Physiology or Medicine; co-discoverer of telomerase
Our genetic material has remarkable structures called telomeres that protect it at the chromosomal level. These special regions shield our genomic material with repeating DNA sequences. Let’s learn about how these molecular guardians work and their role in cellular aging.
Structure of telomeres: TTAGGG repeats and Shelterin complex
The DNA in mammalian telomeres has a repeating sequence TTAGGG. Humans have 9-15 kilobases of this sequence while rodents can have up to 100 kilobases [1]. This structure serves a specific purpose – it creates protective caps at chromosome ends. The telomere ends with a unique feature: a 50-300 nucleotide single-stranded protrusion from the 3′ end, known as the G-overhang or G-tail [2].
The G-rich overhang can bend back and merge into the telomere’s double-stranded region. This creates what scientists call a t-loop structure. The t-loop works like a protective knot that hides the chromosome end, which prevents it from being seen as damaged DNA [2]. The 3′ overhang pairs with the C-rich strand in this structure and pushes the G-rich strand into what’s called a D-loop [3].
A specialized protein complex called shelterin helps protect and maintain telomeres. This six-protein group has:
- TRF1 and TRF2: Bind specifically to double-stranded telomeric DNA
- POT1: Attaches to the single-stranded overhang
- RAP1, TIN2, and TPP1: Bridge the DNA-binding modules and are vital for chromosome end protection [1]
TRF2 helps build t-loops and stops DNA damage responses. TRF1 reshapes DNA to help telomeres replicate efficiently [1].
Function in chromosomal stability and cell division
Telomeres act as vital protectors of genomic stability. They stop chromosomes from breaking down, combining unnecessarily, or fusing with other chromosomes [4]. Without this protection, the cell would mistake chromosome ends for broken DNA and try to repair them by fusing them together.
Telomeres also help solve the “end replication problem.” DNA polymerase can’t fully copy the lagging strand during DNA replication, which would make chromosomes shorter after each division [4]. The specialized structure of telomeres also protects the ends from breaking down [4].
Healthy telomeres prevent DNA damage checkpoints and double-strand break repair pathways from activating [2]. TRF2 guards chromosome ends by controlling the single-stranded 3′ overhang formation. POT1 helps protect the ends by supporting t-loop formation [2].
Why do telomeres shorten with age?
Telomeres wear down over time despite their protective role. Each time a normal human cell divides, it loses 50-200 base pairs of telomeric DNA [2][5]. Two main factors cause this shortening:
The “end replication problem” means DNA polymerase can’t completely copy the 3′ end during cell division [4][5]. The G-rich sequence in telomeres makes them very vulnerable to oxidative damage [5].
Human liver tissue loses about 55 base pairs of telomeric DNA yearly [4]. Telomere length in humans decreases by 24.8–27.7 base pairs each year [4].
Cells stop dividing and enter replicative senescence when telomeres become too short [4]. This natural limit on cell division helps prevent tumors from forming [1]. Lifestyle choices like smoking, obesity, lack of exercise, and poor diet can speed up telomere shortening and lead to early aging or health problems [4].
Specific markers of telomere problems, like stathmin and EF-1a, become more common as people age and develop age-related diseases [4]. Telomere length has a negative relationship with p16, a protein that increases in aging cells [4].
Our knowledge of connection between telomere length and aging opens new possibilities to address age-related diseases and maybe even slow down aging itself.
Further resources:
- National Human Genome Research Institute: https://www.genome.gov/genetics-glossary/Telomere
- Nature Reviews Molecular Cell Biology: https://www.nature.com/nrm
- Cell and Bioscience Journal: https://cellandbioscience.biomedcentral.com
How Telomerase Maintains Telomere Length
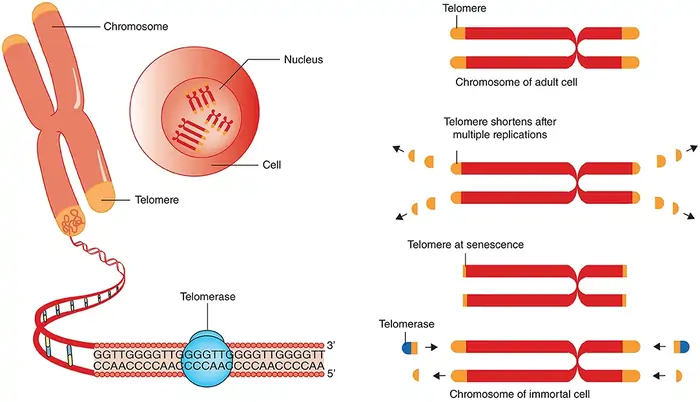
Image Source: Frontiers
Most cells experience telomere shortening with each division. However, some cells have a remarkable enzyme that rebuilds these vital chromosome caps. Scientists call telomerase the “immortality enzyme” because it solves the end replication problem by adding telomeric repeats to chromosome ends.
Telomerase components: TERT and TERC
Telomerase works as a specialized ribonucleoprotein complex with two main parts. The protein subunit telomerase reverse transcriptase (TERT) comes from the hTERT gene on chromosome 5p15.33. The RNA component (TERC or TR) comes from the TERC gene on chromosome 3q26.3 [6]. These components work together in an intricate way. TERT handles DNA synthesis while TERC provides the RNA template that TERT uses to make telomeric TTAGGG repeats [7].
Of course, the active telomerase holoenzyme needs more than just these two parts. It also has:
- The dyskerin-NOP10-NHP2-GAR1 tetramer (known as the H/ACA RNP complex) that helps in telomerase RNA biogenesis
- TCAB1 (also known as WRAP53), which brings telomerase to subnuclear Cajal bodies
- The histone proteins H2A and H2B, which stabilize critical TERT-interacting domains [8]
Building this functional telomerase complex requires RNA maturation, protein assembly, and movement within the nucleus. TERT and TERC can create telomerase activity in vitro, but the other components are vital in living cells [6].
Telomerase activity in stem cells vs somatic cells
Different cell types show vastly different telomerase patterns, which creates key differences in how they maintain telomeres. Most human somatic cells lose telomerase activity after birth. This leads to shorter telomeres with each cell division [3]. When telomeres become too short, cells either age or die.
Cells that need to divide frequently keep their telomerase active. Embryonic stem cells show high telomerase activity, which keeps their telomeres long and lets them divide indefinitely [3]. Adult stem cells have less telomerase than embryonic ones but enough to slow telomere loss and extend their lifespan [3].
Telomerase expression follows a clear biological pattern:
- High activity in germline cells keeps telomere length across generations
- Moderate activity in stem cells extends division capacity without making cells immortal
- Little or no activity in most somatic cells limits how much they can divide [3]
TERT expression controls this regulation because it limits how much telomerase cells can make. TERC appears in most cells regardless of telomerase activity, which shows that TERT controls how telomerase works [3].
Cancer risk from telomerase overexpression
Telomerase lets cells divide more than usual, so it makes sense that problems with it lead to cancer. In fact, about 90% of human cancers show telomerase reactivation [8]. This makes it one of cancer’s most common molecular changes.
Cancer cells use extra telomerase in several ways. They mainly use it to maintain telomere length, which helps them avoid normal limits on cell division [6]. Without this maintenance, cancer cells would eventually hit a crisis point where unstable genes would kill them.
Telomerase helps cancer cells in other ways too. To cite an instance, see how it affects gene expression, cell growth, survival, and various cell signals [8]. These extra functions might play a big role in cancer development.
Cancer cells turn up telomerase through several methods:
- More copies of TERT and TERC genes [6]
- Changes in the TERT promoter that create new spots for transcription factors [8]
- Different levels of regulatory proteins like c-Myc that control TERT transcription [8]
Most normal tissues have very little telomerase while cancer cells have lots. This difference makes telomerase a promising target for cancer treatments. New inhibitors could stop cancer cells from being immortal while leaving normal cells alone [3].
Further resources:
- National Cancer Institute: https://www.cancer.gov/about-cancer/causes-prevention/risk/myths/antiperspirants-fact-sheet
- Telomere Research Network: https://trn.tulane.edu
- Nature Reviews Cancer: https://www.nature.com/nrc
Methods for Measuring Telomere Length
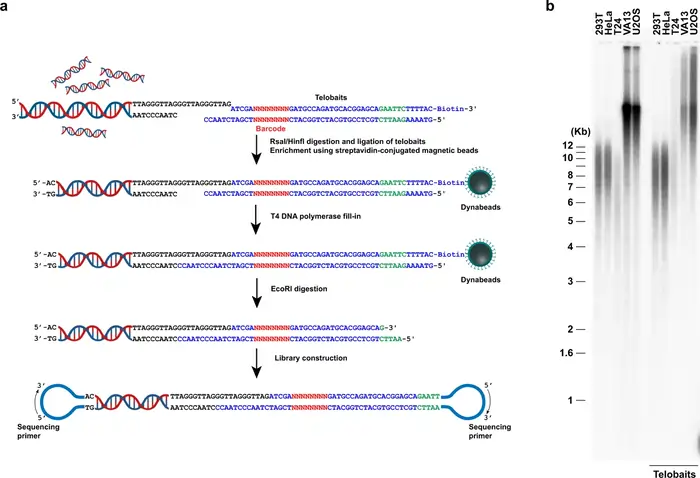
Image Source: Nature
Scientists face many challenges when they try to measure telomere length accurately in aging and disease research. Our understanding of telomere biology has grown, and researchers now use several methods. Each method has its own strengths and weaknesses.
qPCR: high-throughput but variable
Quantitative PCR (qPCR) has become accessible to more people as a method to measure telomeres in large population studies. The method needs minimal DNA (approximately 20 ng per assay) [8]. This technique measures the ratio of telomeric DNA signal (T) to a single-copy gene signal (S). The resulting T/S ratio shows average telomere length [8].
The method gives researchers great advantages in epidemiological research:
- Affordable compared to other techniques
- High-throughput capacity to analyze hundreds or thousands of samples
- Needs nowhere near as much DNA as other methods
Notwithstanding that, qPCR has some drawbacks. Direct comparison studies showed modest correlation with TRF analysis for healthy individuals (R²=0.35) and poor correlation for patients with telomeropathies (R²=0.20) [1]. qPCR’s inter-assay coefficient of variation (16±19.5%) is almost double that of Flow-FISH (9.6±7.6%) [9]. The sensitivity and specificity to detect telomere length below the tenth percentile were much lower for qPCR (40% and 63%) compared to Flow-FISH (80% and 85%) [1].
Flow FISH: gold standard for accuracy
Flow cytometry combined with fluorescence in situ hybridization (Flow-FISH) stands out as the most accurate clinical method to measure telomeres. The technique uses a fluorescent telomere-specific peptide nucleic acid (PNA) probe that binds to telomeric repeats. Scientists then measure these through flow cytometry [10].
Flow-FISH’s value comes from knowing how to analyze telomere length in different leukocyte subpopulations at once from a single blood sample [11]. The method showed excellent agreement with TRF analysis in both healthy individuals (R²=0.60) and patients (R²=0.51) [9].
Scientists have verified this technique as a clinical diagnostic tool under Clinical Laboratory Improvement Amendments (CLIA) certification, especially when diagnosing telomere biology disorders [8]. Detailed comparisons showed Flow-FISH is “more accurate, reproducible, sensitive, and specific in the measurement of human leukocyte’s telomere length” than qPCR [1].
Southern blot and TRF: historical context
Terminal Restriction Fragment (TRF) analysis via Southern blotting remains the original “gold standard” to measure telomeres [12]. Scientists first digest genomic DNA with restriction enzymes that don’t cut telomeric sequences. They then separate these fragments by gel electrophoresis and visualize them using a telomere-specific probe [13].
TRF analysis gives absolute telomere length values in kilobases and shows the entire distribution of telomere lengths in a sample [14]. The method’s precision is remarkable with a coefficient of variation of just 1.74% compared to qPCR’s 6.45% [4].
The method has some critical limitations that pushed researchers toward other techniques:
- Needs large amounts of DNA (approximately 3 μg per sample) [5]
- Takes time and effort (a skilled technician can process about 130 samples weekly) [5]
- Has non-canonical subtelomeric DNA in measurements that might overestimate true telomere length by 1-3 kilobases [15]
Each measurement technique helps us learn about telomere biology differently. Scientists choose methods based on their study’s goals, available samples, and needed accuracy. Flow-FISH currently gives the best balance of precision and practicality for clinical diagnostics. qPCR remains essential for large-scale epidemiological research despite its limitations.
Further resources:
- National Institute on Aging: https://www.nia.nih.gov/health/genetics-aging
- American Federation for Aging Research: https://www.afar.org/
- Telomere Research Network: https://trn.tulane.edu
Epigenetic Clocks vs Telomere Length
Scientists have found a biological timekeeper beyond physical telomere shortening: epigenetic clocks. These clocks measure DNA methylation patterns at specific CpG sites across the genome. This offers a fresh view on aging at the molecular level.
Overview of DNAmAge, GrimAge, PhenoAge, Hannum, SkinBlood
Epigenetic clocks are different ways to measure biological age through DNA methylation patterns:
Horvath’s DNAmAge (Pan-Tissue Clock): This trailblazing clock predicts chronological age in samples from multiple organs [1].
Hannum’s Clock: The clock uses 71 CpG sites from whole blood samples and shows a remarkable 0.96 correlation with chronological age [2].
SkinBlood Clock: The clock excels at age prediction in skin and blood tissues and shows high correlation with DNAmAge (r = 0.91) [3].
PhenoAge: This advanced clock goes beyond chronological age. It has nine age-related clinical measures to better predict health outcomes and mortality [16].
GrimAge: This newest clock generation has DNA methylation-based surrogate markers for seven plasma proteins and smoking pack-years. It better predicts lifespan and health span [16].
How do telomeres work differently from methylation clocks?
Telomeres and epigenetic clocks capture different aspects of aging. These biological mechanisms work in unique ways:
Telomeres measure physical chromosome deterioration from cell division and oxidative damage. Scientists call this “mitotic age”—the total history of cell divisions in tissue [1]. They show genomic integrity and remaining cellular replicative potential.
DNA methylation patterns change in epigenetic clocks. These chemical modifications affect gene expression without changing the genetic sequence. Environmental exposures, lifestyle factors, and intrinsic aging processes leave their mark on these patterns [1].
Studies confirm that epigenetic age and telomere attrition use independent genes with no functional overlap [1]. Epigenetic clocks reveal lifestyle habits and environmental factors that telomeres miss [1]. DNA methylation data identifies biological damage from factors like cigarette smoking that telomere measurements might not catch [1].
Correlation between telomere length and epigenetic age
Telomere length and epigenetic age show modest relationships despite measuring different aging aspects:
Research shows significant inverse correlations between leukocyte telomere length and epigenetic age using PhenoAge, GrimAge, and Hannum clocks [3]. DNAmAge and SkinBlood clock correlations weren’t statistically significant after adjusting for age, sex, and race [3].
These correlations range from modest to moderate. Flow-FISH telomere length measurements correlated with PhenoAge (-0.49), Hannum (-0.43), and GrimAge (-0.41) [3]. Shorter telomeres usually match more advanced epigenetic age. The relationship isn’t strong enough to make them interchangeable.
Telomere length associates with immune-related aging measures (EEAA) but not immune-independent ones (IEAA) [3]. This suggests telomeres might better reflect immune system aging than tissue-independent aging.
Both markers work well together to predict chronological age. One study showed telomere length explained 6.6% of age variance and epigenetic clock explained 19.8%. Together they explained 25.9%, which suggests they capture different aging aspects [17].
Further resources:
- National Institute on Aging: https://www.nia.nih.gov/health/epigenetics-aging
- Telomere Research Network: https://trn.tulane.edu
- Nature Reviews Molecular Cell Biology: https://www.nature.com/nrm
Intrinsic vs Extrinsic Age Acceleration
DNA can tell us how fast we age compared to our actual years. Scientists measure this through epigenetic age acceleration. They use two different methods that show unique aspects of cellular aging.
IEAA: immune-independent aging
Scientists calculate intrinsic epigenetic age acceleration (IEAA) by looking at how cells age without considering changes in blood cell counts [6]. They use complex calculations that compare DNA methylation age against chronological age and blood cell estimates [6].
IEAA reveals basic aging processes that remain consistent in different types of cells and organs [6]. The unique aspect of IEAA is that it shows cellular aging without immune system influences. Your cells’ aging process stands alone here.
Research shows IEAA doesn’t connect with lifestyle choices [7]. This suggests genetic factors might control this type of aging more strongly. The measure stays consistent in cells of all types [7], which reinforces its role in showing how cells naturally age.
EEAA: immune-related aging and telomere correlation
Extrinsic epigenetic age acceleration (EEAA) takes a wider view of biological aging. Scientists calculate it using 71 specific CpGs from the Hannum clock and combine this with three blood cell types: naïve cytotoxic T cells, exhausted cytotoxic T cells, and plasmablasts [6].
EEAA shows positive links to exhausted CD8+ T cells and plasma B cells. It also shows negative links to naïve CD8+ T cells [18]. This means EEAA tracks both blood cell composition changes and internal epigenetic shifts as we age [19].
EEAA becomes even more valuable because it relates to telomere length. Research shows strong negative correlations between EEAA and telomere length measured through flow-FISH (r = −0.32) and qPCR (r = −0.20) [3]. These relationships hold true even after accounting for sex and race [3].
Why telomeres line up more with EEAA
Telomeres and EEAA share a stronger connection than IEAA for good reasons. Telomere shortening and immune aging follow similar biological paths. Both show how cell division and oxidative stress affect our aging immune system.
Short telomeres affect how immune cells work. As telomeres get shorter, immune cells lose their ability to multiply [20]. T cells show this clearly – they temporarily increase telomerase when stimulated but lose this ability after repeated stimulations [20].
The biological evidence makes a strong case:
- Longer telomeres mean more naïve T cells, as expected [7]
- EEAA and telomere length both show immune system aging [3]
- Naïve T cells decrease and memory T cells increase as telomeres shorten with age [19]
These findings show how telomere length and EEAA work together to teach us about immune system aging in ways IEAA cannot. Both help predict overall mortality [19], likely because they record different immune responses to various environmental conditions.
Further resources:
- National Institute on Aging: https://www.nia.nih.gov/health/what-do-we-know-about-healthy-aging
- American Federation for Aging Research: https://www.afar.org/
- Telomere Research Network: https://trn.tulane.edu
Genetic and Environmental Influences on Telomere Length
“You have the power to influence whether your telomeres are going to shorten early, or whether they are going to stay supported and healthy.”
— Dr. Elissa Epel, Professor of Psychiatry, University of California, San Francisco; co-author of ‘The Telomere Effect’
Time isn’t the only factor that determines our telomere length—we inherit it from our parents, and our environment plays a vital role in shaping these biological timepieces.
Heritability and parental age effects
Studies of twins and families show that telomere length runs strongly in families, with inheritance rates ranging from 34% to 82% across different populations [21]. A complete meta-analysis found this inheritance rate at about 70% [21]. Mothers pass on telomere length more strongly than fathers do—the connection between mother and child (r=0.42) is stronger than father and child (r=0.33) [21].
The age of parents when having children affects their offspring’s telomere length. Older fathers tend to have children with longer telomeres, and research shows a positive link between father’s age and telomere length (β=0.005) [21]. This “paternal age effect” stays true even when considering the mother’s age [21]. One reason could be that sperm telomerase activity gets higher as fathers age, which leads to longer telomeres being passed down.
Impact of stress, smoking, and lifestyle
Your lifestyle can affect your telomeres by a lot. Smoking damages telomeres—people who grew up with parents who smoked had shorter telomeres in nearly all their cells [8]. A father’s smoking habits hurt telomeres more than a mother’s [8], though both parents’ smoking added up to make telomeres even shorter.
Your body shape matters too. Higher BMI and bigger waist size link to shorter telomeres [8]. Regular exercise helps keep telomeres healthy, while sitting around too much makes them shorter faster [22].
Socioeconomic status and early-life adversity
Early-life money and social status leave lasting marks on telomere biology. Kids born to mothers with less education had telomeres 3.3% shorter than those whose mothers were highly educated [23]. A mother’s job type linked to their child’s telomere length—children whose mothers had lower-ranked jobs showed 4.0-4.3% shorter telomeres [23].
Tough childhood experiences speed up cellular aging. Trauma, neglect, and stress in childhood make telomeres shorter faster [24]. The more hardships someone faces, the shorter their telomeres become [24].
Having good social support helps protect cells. Strong family relationships link to longer telomeres [25], which suggests that caring relationships might reduce the effects of early hardships on cellular aging.
Further resources:
- National Human Genome Research Institute: https://www.genome.gov
- Centers for Disease Control: https://www.cdc.gov/aging
- American Federation for Aging Research: https://www.afar.org
Limitations of Telomere Length as a Biomarker
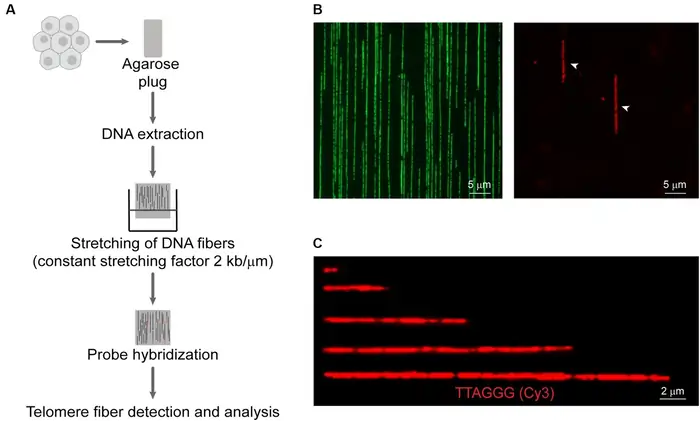
Image Source: Frontiers
Telomeres give us great insights into how cells age, but their use as definitive biomarkers comes with several key limitations. Scientists need to understand these constraints to analyze telomere data with better accuracy.
High inter-individual variability
People’s telomere lengths show remarkable differences right from birth and throughout their lives [26]. These differences make it hard to set normal ranges or risk thresholds for diseases. Research shows that leukocyte telomere length (LTL) varies widely among newborns [26] and adults show this same pattern of high variation [27]. The original phase of telomere shortening happens faster in early life for both humans and non-human primates. This process slows down once we reach adulthood [26].
Genetic factors play a big role in these differences. All genome-wide variants explain about 8.1% of LTL heritability [28]. Twin and family studies point to much higher overall inheritance, which tells us the patterns are complex.
Tissue-specific differences in telomere length
Most telomere studies look at blood leukocytes and miss out on cell-type and tissue-specific variations [8]. Scientists analyzed post-mortem tissues and found major differences in telomere length between tissue types [8]. Cell types within the same tissue showed unique telomere patterns [8].
Whole blood telomere measurements relate to other tissues’ measurements and show positive links with 15 out of 23 tissue types. These relationships aren’t very strong though—they range from 0.15 to 0.37 [29]. Blood telomere length explains only 2% (testis) to 14% (tibial nerve) of telomere length variation in other tissues [29].
Leukocyte composition and immune turnover
Blood contains different types of leukocytes with unique replication histories [27]. Mature myeloid cells rarely divide, but lymphocytes divide many times. This creates big variations within a single blood sample [27]. Naïve T cells have longer telomeres than memory T cells. CD28+ cells show longer telomeres than more differentiated CD28− CD8+ T cells [27].
These differences mean average telomere measurements might miss crucial information about specific disease-related cells. The adaptive immune response targets specific antigens, so average telomere length can’t predict the telomere length of antigen-reactive lymphocytes. These make up just a small part of total lymphocytes [27].
Further resources:
- National Human Genome Research Institute: https://www.genome.gov
- American Federation for Aging Research: https://www.afar.org
- Telomere Research Network: https://trn.tulane.edu
Complementary Use of Telomeres and Epigenetic Clocks
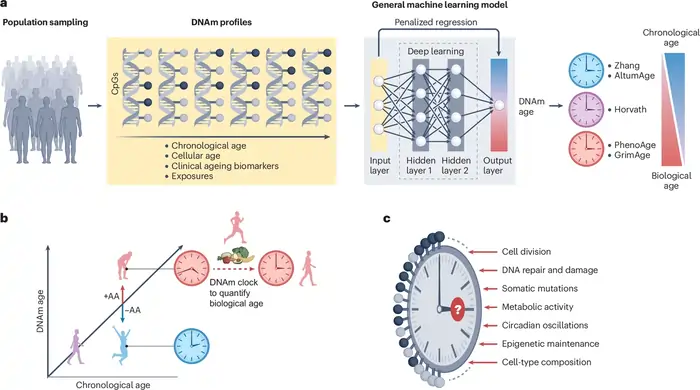
Image Source: Nature
Scientists now recognize that using multiple aging biomarkers together works better than single measurements. A combination of telomere length measurements and epigenetic age calculations provides deeper insights into how we age.
Why combining biomarkers gives better aging estimates
Scientists analyzed both telomere length and epigenetic clocks and found these different biological aging measures showed surprisingly little agreement [30]. The combined predictive power of these markers is a big deal as it means more accuracy than either one alone. A recent analysis showed telomere length explained 6.6% of age variance, while Hannum epigenetic age explained 19.8%. Together, they accounted for 25.9% [2]. This complementary relationship exists because each measure captures unique aspects of aging that work independently [31].
Use in disease prediction and clinical trials
These biomarkers show remarkable predictive capabilities together:
Mortality risk: Combined analyzes revealed each standard deviation increase in baseline epigenetic age linked to a 25% higher mortality risk. Each standard deviation increase in baseline telomere length independently showed an 11% lower mortality risk [2].
Cardiovascular outcomes: DNAmTL (DNA methylation-based telomere length) performed better than traditional leukocyte telomere length at predicting time-to-coronary heart disease (HR=0.55) and time-to-congestive heart failure (HR=0.32) [32].
Frailty measurement: Epigenetic age acceleration shows a clear link to frailty measured by deficit accumulation approaches. This translates to roughly half an additional deficit per 6 years of age acceleration [31].
Future directions in personalized aging diagnostics
Standardization remains the biggest problem – comparing data from different research settings becomes difficult without defined techniques [33]. Scientists believe using both telomere measurements and epigenetic clocks could help identify ways to slow down biological aging.
Clinical applications now go beyond assessing disease risk to monitor how well interventions work. These combined biomarkers could speed up drug development for age-related diseases by serving as surrogate endpoints in clinical trials, eliminating the need to wait decades for clinical outcomes [1].
Further resources:
- National Institute on Aging: https://www.nia.nih.gov/health/what-do-we-know-about-healthy-aging
- American Federation for Aging Research: https://www.afar.org/
- Telomere Research Network: https://trn.tulane.edu
Telomere Length: What It Reveals About Your Health
Telomere length varies significantly between individuals and can serve as a marker of overall health status and disease risk.
Normal Telomere Length Ranges
Telomere length is typically measured in kilobases (kb) or as a ratio compared to a single-copy gene. Average telomere length decreases throughout life:
- Newborns: 8-13 kb
- Young adults: 7-9 kb
- Middle-aged adults: 6-8 kb
- Elderly (85+): 4-6 kb
However, these ranges show considerable variation between individuals of the same age. Studies of twins suggest that while approximately 30% of telomere length is determined by genetics, the remaining 70% is influenced by environmental and lifestyle factors.
What Short Telomeres May Indicate
If your telomeres are significantly shorter than average for your age group, it may indicate:
- Accelerated cellular aging
- Higher oxidative stress and inflammation levels
- Increased risk of age-related diseases
- Potential exposure to significant life stressors
- Lifestyle factors that negatively impact cellular health
Research from the University of California found that individuals whose telomeres were 10% shorter than their age-matched peers had approximately a 23% higher mortality risk over the following 10-15 years.
Beyond Length: Telomere Health Markers
Scientists are now looking beyond simple telomere length to assess telomere health more comprehensively:
- Telomere shortening rate: How quickly telomeres shorten over time
- Telomere damage: DNA damage specifically within the telomere region
- Shelterin protein levels: The abundance of protective proteins at telomeres
- Telomerase activity: Levels of the enzyme that can rebuild telomeres
These markers collectively provide a more nuanced picture of telomere biology and its impact on health.
Factors That Accelerate Telomere Shortening
Multiple lifestyle and environmental factors can speed up telomere shortening, potentially accelerating biological aging.
Psychological Stress
Chronic psychological stress has been consistently linked to accelerated telomere shortening:
- A groundbreaking study by Dr. Blackburn and Dr. Elissa Epel found that mothers caring for chronically ill children had telomeres that appeared 9-17 years older than their chronological age.
- Each major life stressor is associated with an additional year of telomere aging, according to research from the University of California, San Francisco.
- Work-related stress, particularly when combined with poor recovery time, correlates with shorter telomeres.
The mechanism appears to involve stress hormones like cortisol, which increase oxidative stress and inflammation, both of which can damage telomeres.
Poor Diet
Dietary patterns significantly impact telomere health:
- Processed foods: High consumption of ultra-processed foods correlates with shorter telomeres
- Sugar consumption: Research shows that each daily serving of sugar-sweetened beverages is associated with 4.6 years of additional telomere aging
- Red meat: Regular consumption of processed and red meats has been linked to faster telomere shortening
- Low antioxidant intake: Insufficient consumption of fruits and vegetables deprives cells of protective compounds
A Mediterranean diet, rich in vegetables, fruits, whole grains, fish, and olive oil, has been associated with longer telomeres in multiple studies.
Sedentary Lifestyle
Physical inactivity contributes to faster telomere shortening:
- Sedentary individuals have telomeres that appear up to 10 years older than physically active peers
- Even sitting for prolonged periods has been associated with shorter telomeres
- The effect appears dose-dependent – the more sedentary the lifestyle, the greater the impact on telomere length
Environmental Toxins
Exposure to various environmental toxins accelerates telomere shortening:
- Air pollution: Long-term exposure to particulate matter correlates with shorter telomeres
- Smoking: Cigarette smoking causes an estimated additional 5 years of telomere aging
- Heavy metals: Exposure to lead, mercury, and cadmium has been linked to accelerated telomere shortening
Pesticides: Occupational exposure to certain agricultural chemicals correlates with shorter telomeres
Poor Sleep Quality
Disrupted or insufficient sleep negatively impacts telomere health:
- People sleeping less than 6 hours per night show telomeres comparable to those 9 years older
- Sleep apnea is associated with significantly shorter telomeres
- Shift work that disrupts circadian rhythms accelerates telomere shortening
How to Protect and Support Your Telomeres
While telomere shortening is a natural process, research suggests several evidence-based strategies that may help preserve telomere length and potentially slow biological aging.
Nutrition Strategies for Telomere Health
Research has identified specific dietary patterns and nutrients that may support telomere length:
- Adopt a Mediterranean or plant-forward diet: Rich in vegetables, fruits, whole grains, legumes, and healthy fats A 2018 meta-analysis found adherence to a Mediterranean diet was associated with longer telomeres
Increase antioxidant-rich foods:
- Berries (especially blueberries)
- Dark leafy greens
- Colorful vegetables
- Green tea (3+ cups daily associated with longer telomeres)
Consume omega-3 fatty acids:
- Higher blood levels of omega-3s correlate with slower telomere shortening
- Sources include fatty fish, walnuts, flaxseeds, and chia seeds
- Consider a high-quality supplement if dietary intake is insufficient
Specific nutrients linked to telomere health:
- Vitamin D: Higher levels associated with longer telomeres
- Folate: Crucial for DNA methylation and telomere maintenance
- Vitamin B12: Essential for DNA synthesis and repair
- Zinc: Required for telomere maintenance proteins
Physical Activity for Telomere Protection
Exercise has shown consistent benefits for telomere health:
- Regular moderate exercise: 30-45 minutes of moderate activity most days shows the strongest association with telomere maintenance
- A study in the journal Medicine & Science in Sports & Exercise found physically active individuals had telomeres equivalent to those 9 years younger than sedentary peers
Resistance training:
- Preserves muscle mass and function
- 2-3 sessions weekly associated with better telomere length preservation
Avoid excessive high-intensity exercise:
- Extremely intense, prolonged exercise (like ultramarathons) may actually increase oxidative stress
- Balance high-intensity work with adequate recovery
Stress Management Techniques
Given the strong connection between psychological stress and telomere shortening, stress management is crucial:
- Meditation and mindfulness: An 8-week mindfulness program increased telomerase activity by 43% in one study
- Regular meditation practice is associated with preserved telomere length
Yoga and tai chi:
- Combine physical activity with mindfulness
- 12-week yoga programs have shown improvements in markers of cellular aging
Social connection:
- Strong social relationships buffer against telomere shortening
- Social isolation accelerates cellular aging
Cognitive strategies:
- Cognitive reappraisal (reframing stressful situations)
- Practicing gratitude and positive psychology techniques
Sleep Optimization
Quality sleep is essential for telomere maintenance:
- Aim for 7-8 hours of quality sleep: Consistent sleep-wake schedule
- Cool, dark sleeping environment
Address sleep disorders:
- Screen for and treat conditions like sleep apnea
- Consider cognitive behavioral therapy for insomnia (CBT-I)
Reduce exposure to blue light before bed:
- Use blue light blocking glasses in the evening
- Minimize screen time 1-2 hours before sleep
Supplements with Research Support
While the primary focus should be on lifestyle foundations, some supplements show promising research for telomere support:
- Omega-3 fatty acids (EPA/DHA): 1-2g daily associated with decreased telomere shortening rate
- Look for molecularly distilled products with third-party testing
Vitamin D:
- Maintain levels between 40-60 ng/mL
- Typical doses of 1000-5000 IU daily depending on baseline levels
Astragalus-based supplements:
- Some evidence for telomerase activation
- Quality and standardization vary significantly between products
NAD+ precursors:
- Nicotinamide Riboside (NR) and Nicotinamide Mononucleotide (NMN)
- Support cellular energy production and DNA repair
- Research on direct telomere effects is preliminary but promising
Note: Always consult with a healthcare provider before starting any supplement regimen, especially if you have underlying health conditions or take medications.
Measuring Telomere Length: Available Testing Options
For those interested in assessing their telomere length, several testing options are available, each with different methodologies and considerations.
Commercial Testing Options
Several companies now offer direct-to-consumer telomere testing:
At-Home Test Kits
Test Provider | Method | Sample Type | Price Range | Information Provided |
TeloYears | qPCR | Blood spot | $89-$129 | Telomere length, age comparison |
SpectraCell | Flow-FISH | Blood draw | $290-$350 | Cell-specific telomere measurements |
Life Length | TAT | Blood draw | $350-$500 | Percentage of critically short telomeres |
When selecting a test, consider:
- Test methodology and accuracy
- Whether results include actionable recommendations
- Privacy policies regarding your genetic data
- Whether repeat testing is available to track changes over time
Clinical Laboratory Testing
In some cases, telomere testing may be available through healthcare providers:
- Usually only covered by insurance for diagnosing specific telomere-related disorders
- Typically uses more comprehensive methods than consumer tests
- Often includes expert interpretation of results
- May be combined with other biomarkers of aging
Testing Methodologies
Common methods for measuring telomere length include:
- qPCR (Quantitative Polymerase Chain Reaction): Most common in commercial testing
- Relatively inexpensive
- Measures average telomere length relative to a single-copy gene
- Less precise than some laboratory methods
Flow-FISH (Flow Cytometry with Fluorescence In Situ Hybridization):
- More precise than qPCR
- Can measure telomere length in specific cell types
- Requires specialized equipment
- Generally more expensive
TRF (Terminal Restriction Fragment):
- Considered the gold standard in research
- Measures absolute telomere length
- Labor-intensive and expensive
- Not widely available for consumer testing
TAT (Telomere Analysis Technology):
- Measures distribution of telomere lengths
- Identifies percentage of critically short telomeres
- Requires specialized equipment and expertise
Interpreting Test Results
When reviewing telomere test results, consider:
- Comparison to age-matched peers: How your telomere length compares to others in your age group
- Distribution of telomere lengths: The percentage of very short telomeres may be more important than the average length
- Cell type specificity: Telomere length varies between different types of cells
- Rate of change: A single measurement provides less information than tracking changes over time
Remember that telomere length is just one of many biomarkers of aging and should be interpreted in the context of overall health status and other metrics.
PlexusDx Longevity & Healthy Aging Genetic Test
For those who want a simple, at-home way to track their biological age, the Longevity Telomere Test Kit available on Amazon is a great option. It not only measures your telomere length, which is a key indicator of cellular aging, but also provides personalized supplement and lifestyle recommendations based on your results. It’s easy to use, non-invasive, and gives you practical insights into how well your body is aging — all without leaving your home.
Conclusion
Our deep dive into telomeres reveals these remarkable chromosome caps that act as markers of our biological clock. Age, cell division, and environmental stressors naturally shorten telomeres. Once critically shortened, they trigger cellular senescence – a process linked to many age-related diseases and mortality risk.
Scientists often call telomerase the “immortality enzyme.” It works against telomere shortening, though the body carefully controls its activity to prevent cancer risk. Different measurement techniques like qPCR, Flow-FISH, and TRF analysis each have their strengths and limits, which shows how complex telomere biology can be.
Telomeres tell just one part of our aging story. DNA methylation patterns give us another perspective through epigenetic clocks. Different clocks like DNAmAge, GrimAge, and PhenoAge each show unique aspects of biological aging. The difference between intrinsic (IEAA) and extrinsic (EEAA) epigenetic age acceleration shows how aging follows various biological paths.
Both genes and lifestyle substantially affect telomere health beyond age alone. Your parents’ age, smoking habits, physical activity, and early-life hardships all leave their mark on these biological timekeepers. All the same, high individual variation, tissue-specific differences, and white blood cell composition make it hard to use telomere length as a single aging marker.
The best path forward combines telomere measurements with epigenetic clocks. This approach helps better predict disease risk, mortality, and how well treatments work. Research continues to uncover new details about cellular aging. You might want to learn more about Molecular Mechanisms of Cellular Aging at Longevity Blueprint Health’s latest research on key molecular pathways.
These biological markers are a great way to understand how we age, even with their limits. Learning about them helps us make smart lifestyle choices that could improve our cellular health and possibly extend our healthspan. The science keeps moving forward, giving hope that we might slow biological aging and reduce age-related diseases.
Further resources:
- National Institute on Aging: https://www.nia.nih.gov/health/what-do-we-know-about-healthy-aging
- Telomere Research Network: https://trn.tulane.edu
- American Federation for Aging Research: https://www.afar.org/
Disclosure: This post may contain affiliate links. If you purchase through these links, I may earn a small commission at no extra cost to you. I only recommend products I personally trust and believe add value to your health journey.
FAQs
Do longer telomeres make you look younger?
While longer telomeres may contribute to some signs of youthfulness, such as delayed graying of hair, they don’t necessarily make a person look younger overall. In fact, individuals with mutations causing longer telomeres may face increased risks of certain health issues, including benign and cancerous tumors.
What is the biological clock of aging?
The biological clock of aging refers to various molecular markers that can predict a person’s biological age. These include epigenetic clocks, which measure DNA methylation patterns, and telomere length. The difference between predicted biological age and chronological age can indicate the rate of aging, which is associated with health outcomes and mortality risk.
How do telomeres relate to biological age?
Telomeres are considered one of the best biomarkers of aging. As cells divide and organisms age, telomeres naturally shorten. This shortening process is associated with cellular senescence and various age-related diseases. Therefore, telomere length can provide insights into an individual’s biological age and overall health status.
What is the telomere theory of aging?
The telomere theory of aging proposes that the progressive shortening of telomeres leads to cellular senescence, apoptosis, or potential oncogenic transformation. This process affects the health and lifespan of an individual. According to this theory, shorter telomeres are associated with an increased incidence of age-related diseases and reduced survival rates.
Can lifestyle factors influence telomere length?
Yes, various lifestyle factors can impact telomere length. Studies have shown that smoking, obesity, and sedentary behavior can accelerate telomere shortening. Conversely, regular physical activity, stress management, and a healthy diet may help maintain telomere length. However, it’s important to note that telomere length is also influenced by genetic factors and exhibits high variability among individuals.
What is the relationship between telomeres and life expectancy?
While telomere length correlates with mortality risk, the relationship is complex. Studies show that individuals with the shortest telomeres have a 20-40% higher all-cause mortality risk compared to those with the longest telomeres. However, telomere length is just one of many factors influencing lifespan.
Research from the University of Copenhagen found that the rate of telomere shortening may be more predictive of longevity than absolute length at any single time point. Individuals whose telomeres shortened more slowly over time had lower mortality rates, independent of initial telomere length.Can telomeres grow longer?
Yes, telomeres can grow longer under certain circumstances:
1. Through telomerase activity in cells where the enzyme is active
2. In early embryonic development when telomeres are reset
3. In response to certain lifestyle interventions (though increases are typically modest)
4. In cancer cells that have reactivated telomerase
A 2013 study published in The Lancet Oncology found that comprehensive lifestyle changes (plant-based diet, moderate exercise, stress management, and social support) were associated with a modest increase in telomere length over a 5-year period.How accurate are telomere tests?
The accuracy of telomere tests varies considerably:
1. Variability between methods: Different testing approaches can yield varying results
2. Repeat testing variability: The same method may produce different results when repeated
3. Laboratory differences: Results can vary between different testing facilities
4. Cell type considerations: Telomere length differs between cell types, affecting results based on which cells are tested.For consumer testing, qPCR methods typically have a coefficient of variation of 6-7%, meaning results could vary by this percentage if the test were repeated.
Can children inherit short telomeres from parents?
Yes, telomere length is partially heritable:
1. Approximately 30-80% of telomere length variation appears to be genetic
2. Both maternal and paternal telomere length influence offspring telomere length
3. Children can inherit telomere-related gene variants that affect telomere maintenance
4. Some rare conditions called “telomeropathies” result from inherited telomere maintenance defects.Interestingly, a father’s age at conception influences the telomere length of his children – older fathers tend to pass on longer telomeres, likely because telomerase remains active in sperm-producing cells.
Do men and women have different telomere dynamics?
Yes, gender differences in telomeres are well-documented:
1. Women typically have longer telomeres than men of the same age
2. The rate of telomere shortening appears to accelerate in women after menopause
3. Sex hormones influence telomere biology – estrogen may have protective effects
4. X-chromosome inactivation in women may contribute to sex differences in telomere dynamicsThese differences may partially explain the gender gap in longevity, though many other factors contribute as well.
Can stress really shorten telomeres?
Yes, substantial evidence indicates that psychological stress accelerates telomere shortening:
1. Chronic stress increases oxidative damage and inflammation, both of which can damage telomeres
2. Stress hormones like cortisol may directly impair telomere maintenance mechanisms
3. Early life stress appears to have particularly strong effects on telomere length later in life
4. The perception of stress may be as important as objective stressors.A 2020 meta-analysis of 111 studies confirmed a significant association between various forms of psychological stress and shorter telomeres, with an effect size comparable to that of smoking.
What’s the connection between inflammation and telomeres?
Inflammation and telomere health are closely linked:
1. Chronic inflammation creates an environment of increased oxidative stress that can damage telomeres
2. Inflammatory cytokines can directly impair telomerase activity
3. Short telomeres in immune cells may contribute to dysregulated inflammatory responses
4. Anti-inflammatory interventions may help preserve telomere length.This relationship creates a potential feedback loop – inflammation shortens telomeres, and cells with short telomeres produce more inflammatory signals.
Are there any FDA-approved treatments specifically for telomere health?
Currently, there are no FDA-approved pharmaceutical treatments specifically for telomere lengthening or protection in otherwise healthy individuals. However:
1. Danazol (an androgen) has received orphan drug designation for certain telomere biology disorders
2. Several compounds are in clinical trials for telomere-related conditions
3. Various supplements claim to support telomere health, but these are not evaluated or approved by the FDA for such claims
References
[1] – https://onlinelibrary.wiley.com/doi/full/10.1002/agm2.12197
[2] – https://pmc.ncbi.nlm.nih.gov/articles/PMC4864882/
[3] – https://pmc.ncbi.nlm.nih.gov/articles/PMC9213578/
[4] – https://pmc.ncbi.nlm.nih.gov/articles/PMC4292845/
[5] – https://pubmed.ncbi.nlm.nih.gov/21085125/
[6] – https://bmcmedgenomics.biomedcentral.com/articles/10.1186/s12920-019-0585-5
[7] – https://www.nature.com/articles/s41467-017-02697-5
[8] – https://www.nature.com/articles/s42003-022-03521-7
[9] – https://ashpublications.org/blood/article/124/21/1605/88562/Better-Performance-of-Flow-FISH-in-Comparison-to
[10] – https://www.nature.com/articles/s41598-022-27196-6
[11] – https://repeatdx.com/flow-fish/
[12] – https://www.tandfonline.com/doi/full/10.2144/btn-2019-0082
[13] – https://pmc.ncbi.nlm.nih.gov/articles/PMC5784071/
[14] – https://academic.oup.com/nar/article/39/12/e84/2411711
[15] – https://www.nature.com/articles/s41467-024-49007-4
[16] – https://www.sciencedirect.com/science/article/abs/pii/S1568163724002368
[17] – https://academic.oup.com/ije/article/45/2/424/2572710
[18] – https://pmc.ncbi.nlm.nih.gov/articles/PMC5536960/
[19] – https://www.aging-us.com/article/101293/text
[20] – https://immunityageing.biomedcentral.com/articles/10.1186/s12979-022-00273-0
[21] – https://www.nature.com/articles/ejhg2012303
[22] – https://pmc.ncbi.nlm.nih.gov/articles/PMC8745211/
[23] – https://jamanetwork.com/journals/jamanetworkopen/fullarticle/2765374
[24] – https://pmc.ncbi.nlm.nih.gov/articles/PMC5608639/
[25] – https://pubmed.ncbi.nlm.nih.gov/29035711/
[26] – https://pmc.ncbi.nlm.nih.gov/articles/PMC3202050/
[27] – https://pmc.ncbi.nlm.nih.gov/articles/PMC3423542/
[28] – https://www.nature.com/articles/s41588-021-00944-6
[29] – https://pmc.ncbi.nlm.nih.gov/articles/PMC8108546/
[30] – https://pmc.ncbi.nlm.nih.gov/articles/PMC6248475/
[31] – https://clinicalepigeneticsjournal.biomedcentral.com/articles/10.1186/s13148-016-0186-5
[32] – https://www.aging-us.com/article/102173/text
[33] – https://www.sciencedirect.com/science/article/abs/pii/S0024320524004326