Biological Aging: Shocking Molecular Shifts Begin at 30
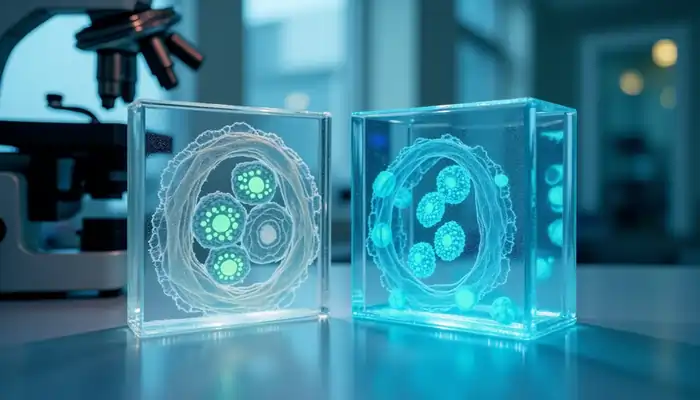
The way we age isn’t as simple as we used to believe. A Stanford Medicine study shows our bodies go through dramatic molecular changes at specific ages, especially around 44 and 60. This remarkable finding challenges everything we knew about growing older.
Our biological aging happens in distinct phases instead of a steady decline. Scientists found something surprising – 81% of the molecules they studied didn’t change in a straight line. While we add one year to our age annually, our body’s biological age can be quite different. To cite an instance, people who do endurance training can reduce their death risk by three to five times compared to those who don’t exercise and improve their longevity health.
These molecular changes in our bodies start when we turn 30. The changes affect us at the cellular level and reshape our understanding of aging. Our DNA repair systems and proteins undergo hidden biological changes that determine how we age.
The Science of Aging: What Happens at the Molecular Level
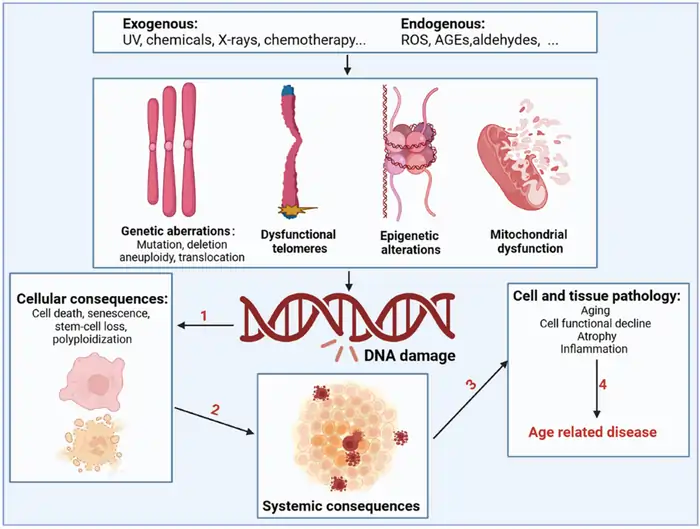
Image Source: Cell Communication and Signaling – BioMed Central
The molecular realm shows how complex aging really is. Scientists who look into our cells find that biological age—how old our bodies look at the molecular level—is different from the chronological age on our birth certificates [1]. This difference is significant to understand why some people age faster or slower than others.
Understanding biological vs. chronological age
Chronological age counts the years we’ve lived, but biological age shows our cells’, tissues’, and organ systems’ actual state based on biochemical markers [2]. Scientists can’t determine biological age with a single test. They need to look at multiple biomarkers like blood tests, heart rate, blood pressure, and DNA methylation patterns [2]. These measurements create a customized picture of aging and longevity health.
The gap between chronological and biological age can be big. Research shows that African Americans experience faster aging, making their biological age about three years greater than their white peers of the same chronological age [1]. Studies also show that childhood intelligence relates to biological aging. People with higher childhood IQ scores tend to have younger biological age measurements as adults [1].
The 9 hallmarks of aging identified by researchers
Scientists identified nine basic processes—called hallmarks—that guide aging across species in 2013 [3]. These hallmarks include:
- Genomic instability (DNA damage accumulation)
- Telomere attrition (shortening of chromosome ends)
- Epigenetic alterations (changes in gene expression)
- Loss of proteostasis (protein quality control failure)
- Deregulated nutrient-sensing (metabolic changes)
- Mitochondrial dysfunction (energy production decline)
- Cellular senescence (zombie-like cells that won’t die)
- Stem cell exhaustion (reduced regenerative capacity)
- Altered intercellular communication (cell signaling changes)
Each hallmark meets three key criteria: it shows up during normal aging, speeds up aging when increased experimentally, and slows down when targeted by interventions [4]. Scientists added three more hallmarks in 2023: disabled macroautophagy (cellular cleanup), chronic inflammation, and dysbiosis (microbiome imbalance) [3].
These hallmarks work together in an interconnected network. Telomere shortening happens naturally with age and works like a biological clock that ticks with each cell division [5]. Mitochondrial dysfunction creates more reactive oxygen species (ROS), which causes additional cellular damage [5].
Why age 30 marks a critical turning point
Age 30 represents a major biological threshold, though aging happens throughout life. Most body systems’ overall performance decreases by about 1% each year after 30 [1]. The body’s metabolic rate slows down too, burning about 12 fewer calories daily for each year after 30 [1].
Several key changes begin at the molecular level around age 30:
- Electrical forces that bind molecules together start getting weaker [1]
- People lose about 6 pounds of muscle mass in the following decade [1]
- Women go through one of their fastest aging phases [1]
A newer study suggests that aging isn’t just a steady decline but has specific speed-up points [1]. The largest longitudinal study that analyzed blood, urine, and fecal samples along with facial dimensions and various molecular markers found that women typically age faster around both age 30 and 50 [1].
These findings challenge what we thought we knew about aging as a gradual process. They highlight why we need preventive measures starting in our early 30s—right when our molecular machinery starts showing its first signs of wear.
Hidden Changes in Your 30s: The Beginning of Cellular Aging
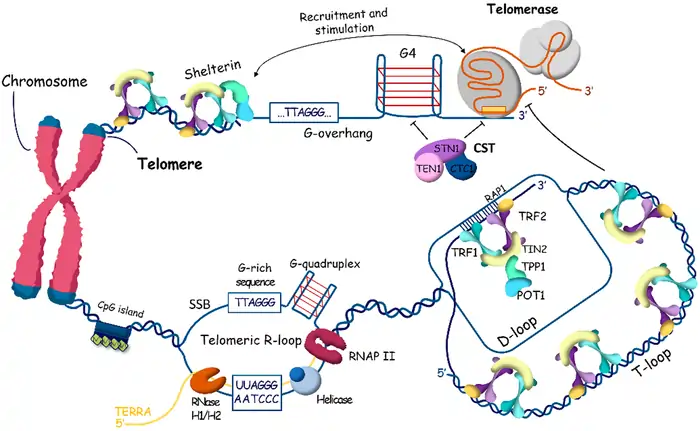
Image Source: MDPI
The cellular machinery in your body starts showing subtle yet important changes by age 30. You can’t see these molecular changes with your eyes, but they mark the start of biological aging in your cells.
In this section:
- Telomere shortening
- Mitochondrial efficiency decline
- DNA repair mechanism slowdown
- Oxidative stress accumulation
Telomere shortening: The biological clock starts ticking
Telomeres are repetitive DNA sequences that act as the “sacrificial anode” of your genetic material [6]. Your cells lose about 50 base pairs in length from these protective caps during each division [6]. This natural process speeds up around age 30 and works like a biological clock for cellular aging.
Scientists have long considered Telomere length (TL) one of the best aging biomarkers [7]. Research now shows that TL by itself gives only a rough estimate of how fast we age [8]. In spite of that, telomere shortening remains crucial among other markers like homeostatic dysregulation indices and epigenetic clocks [8].
Related post: Telomere Length and Aging
Mitochondrial efficiency decline: Why energy levels change
Your cells’ power plants – mitochondria – undergo major efficiency changes in your 30s. Research links aging to a clear drop in mitochondrial capacity, which affects how well you exercise, use your muscles, and process insulin [9].
Older adults show a 17% lower maximum capacity in their electron transport chain [9] and decreased ADP-stimulated respiration [9]. This mitochondrial dysfunction is a big deal as it means that people have 26% less maximum aerobic capacity and weaker muscles compared to younger adults [9].
Related post: The Functions of Mitochondria
The first signs of DNA repair mechanism slowdown
Your DNA repair mechanisms start losing their efficiency around age 30, which makes preventing genetic damage harder [10]. Young adults (age 1-3 months) can fix DNA damage within 6 days, but older individuals repair only about 25% in the same time [11].
Multiple repair pathways slow down at once. Mismatch repair (MMR), base excision repair (BER), nucleotide excision repair (NER), and double-strand break (DSB) repair become less effective with age [12]. DNA damage builds up faster as a result and contributes to cellular dysfunction and aging [11].
Oxidative stress accumulation: The free radical theory
Scientists first proposed the free radical theory of aging in 1956. The theory suggests that reactive oxygen species (ROS) from normal metabolism damage cells over time [2]. These reactive molecules harm proteins, lipids, and DNA when they overwhelm the body’s antioxidant defenses [2].
The balance between ROS production and antioxidant capacity starts shifting in your 30s. Oxidative stress damages mitochondrial DNA 10 times more easily than nuclear DNA [2]. Damaged mitochondria then create a “vicious cycle” by producing even more ROS [13].
The free radical theory isn’t the only explanation for aging [14]. Modern science suggests oxidative damage is just one part of the biological damage that happens as we age [14]. The buildup of oxidative stress remains a major factor in the cellular aging that begins in your 30s.
Resource Links:
- National Institute on Aging: https://www.nia.nih.gov/health/what-do-we-know-about-healthy-aging
- Harvard Health: https://www.health.harvard.edu/topics/aging
- American Federation for Aging Research: https://www.afar.org/
The Aging Cascade: How Molecular Changes Accelerate in Your 40s
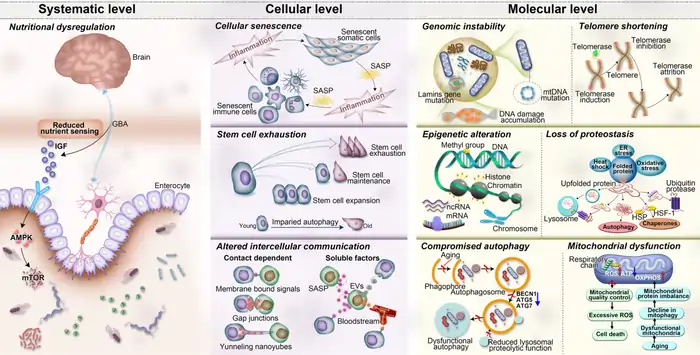
Image Source: Nature
The human aging process reaches a turning point in our mid-40s, according to breakthrough Stanford research. This stage triggers molecular changes that make it a unique biological phase rather than just another decade of life.
In this section:
- The dramatic mid-40s change found by Stanford researchers
- Metabolism and alcohol processing changes
- Cardiovascular molecular markers that emerge
- Why both men and women experience this transition
The dramatic mid-40s change found by Stanford researchers
Stanford Medicine researchers made a remarkable discovery: aging happens in distinct bursts rather than gradually. Their detailed study showed that most molecules and microbes don’t change slowly with age. They undergo rapid changes around ages 44 and 60 [15]. The cluster of changes in the mid-40s surprised scientists with its unexpected importance [3].
Metabolism and alcohol processing changes
Several metabolic changes happen when you reach your 40s. Your resting metabolic rate slows down by about 5% every decade after age 40 [16]. Your metabolism that burns 1,200 calories daily at age 40 will drop to around 1,140 by age 50 [16].
The researchers found the most important changes in alcohol, caffeine, and lipid metabolism [15]. The liver produces fewer enzymes, which means alcohol stays in your system longer [4]. Many people become more sensitive to alcohol in their 40s and react more strongly to the same amount they used to drink [17].
Cardiovascular molecular markers that emerge
Research has identified specific molecular changes linked to cardiovascular disease during this time [15]. The aging heart goes through complex metabolic changes:
- Reduced myocardial lipid catabolism [18]
- Increased anaerobic glycolysis instead of glucose oxidation [18]
- Changes in insulin responsiveness affecting heart function [18]
These markers signal the start of more noticeable changes in cardiovascular health.
Why both men and women experience this transition
Scientists first thought menopause or perimenopause in women might explain the mid-40s transition. The data showed that men experience equally important changes during this time [3]. This suggests that while hormones play a role, more basic biological processes cause this transition in both sexes [15].
Men’s testosterone levels continue to decrease gradually from their 30s. Women start experiencing faster estrogen changes [19]. These hormone changes alone don’t explain the similar molecular patterns seen in both genders.
Resource Links:
- National Institute on Aging: https://www.nia.nih.gov/health/aging-process
- American Federation for Aging Research: https://www.afar.org/
- Stanford Center on Longevity: https://longevity.stanford.edu/
The Science Behind Age-Related Protein Changes
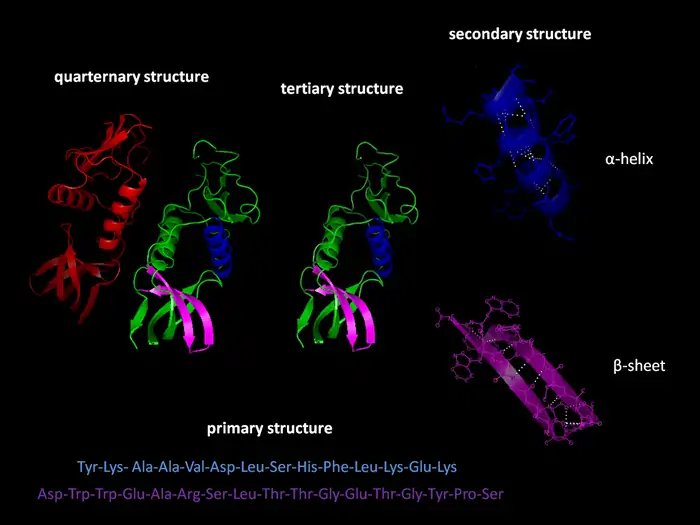
Image Source: Wikipedia
Proteins power our cells’ functions and change dramatically as we get older. Scientists have found that these molecular changes play a bigger role in biological aging than we once thought.
Protein folding errors and their consequences
Our cells need proteins to fold into exact 3D shapes to work properly. This folding process becomes more error-prone with age. Scientists have found that aging tissues contain 1.3 to 2.5 times more insoluble protein clumps than younger samples [1]. These misshapen proteins behave like disease-causing aggregates – they don’t break down with detergents, resist protease enzymes, and stick to amyloid-specific dyes [1].
These folding mistakes affect more than just individual proteins. Misfolded proteins disrupt key cell functions and can both disable normal protein activity and create toxic effects [1]. These protein clumps build up throughout our lives and steadily impair cell function [1].
How proteostasis network efficiency declines
Your body keeps proteins balanced through a complex system called the proteostasis network. This network has molecular chaperones, protein-cutting machinery, and control factors that work together to make, fold, and break down proteins [20].
This vital system weakens with age. The Ubiquitin-Proteasome System (UPS), which removes damaged proteins, becomes less effective over time [1]. Your body also loses its ability to trigger protective responses needed to fix protein damage [21].
This creates a dangerous cycle. Misfolded proteins pile up and overwhelm the proteostasis machinery, which leads to even more protein clumping [1]. Your cells’ quality control system simply can’t keep up.
The role of chaperone proteins in aging
Chaperone proteins guard against protein misfolding. They stop proteins from sticking to things they shouldn’t by blocking their sticky areas [22].
Studies show that specific chaperones like Hsc70 become less active with age [22]. This drop makes it harder for cells to handle misfolded proteins. The chaperone Hsp104 increases until the final cell division, which suggests cells try to fight back against protein problems [23].
The balance between needed and available chaperones gets disrupted as we age. More protein damage and fewer working chaperones create an “overload” situation [24].
Measuring your biological age through protein biomarkers
Scientists now use protein-based “clocks” to measure biological aging. A breakthrough study looked at 2,925 proteins from over 4,200 adults between 18 and 95 years old. They found 373 proteins that together accurately predict age [25].
These protein patterns tell an interesting story. Aging proteins don’t change smoothly but instead shift dramatically at three specific ages: 34, 60, and 78 [25].
Scientists have developed a blood test that uses machine learning to analyze more than 200 proteins. This test estimates biological age and disease risk [26]. The protein clock works accurately for people from different genetic backgrounds and health conditions [27].
Resource Links:
- National Institute on Aging: https://www.nia.nih.gov/
- American Federation for Aging Research: https://www.afar.org/
- Harvard Medical School: https://hms.harvard.edu/
Materials and Methods: How Scientists Study the Biology of Aging
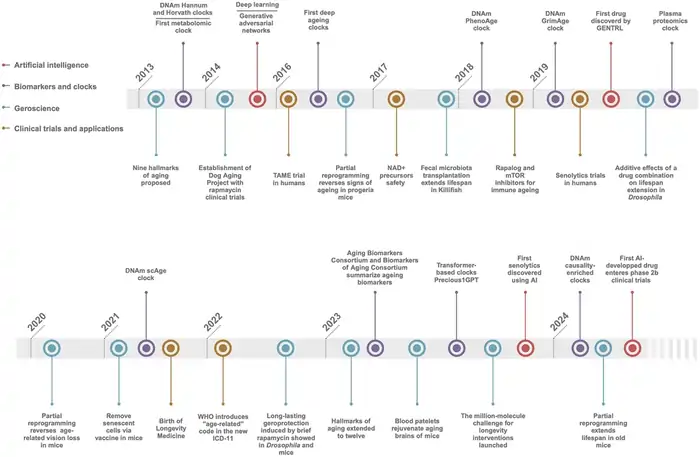
Image Source: BiopharmaTrend
Scientists need sophisticated tools and methodologies to solve the mystery of aging. These methods have changed dramatically in the last few decades. Research teams now use various techniques to discover why and how our bodies age.
Related post: Longevity Science
Longitudinal studies tracking molecular changes
Longitudinal studies give us better insights than cross-sectional research. They don’t just take snapshots – they follow the same people for years. Recent studies that tracked participants for up to 6.8 years (median 1.7 years) have created over 246 billion data points. These come from blood, stool, skin, oral, and nasal samples [28]. Scientists can now detect baseline markers and track individual changes. This method eliminates factors like BMI, sex, and ethnicity that might skew results [28].
A groundbreaking study showed aging isn’t linear at all. The research found 81% of molecular markers follow nonlinear patterns. Only 6.6% showed linear age-related changes [29]. Two major transition periods emerge around ages 44 and 60. These ages mark dramatic transformations in molecular pathways [30].
Biomarker analysis techniques
Scientists calculate biological age through several methods:
- DNA methylation analysis: This looks at epigenetic markers that relate to chronological age within a few years’ precision [5]
- Telomere length assessment: Scientists use Telomere Restriction Fragment (TRF) analysis, Quantitative Fluorescence In Situ Hybridization (Q-FISH), and Flow-FISH [31]
- Senescence-Associated β-galactosidase (SA-β-gal): This tells senescent cells apart from quiescent ones [31]
- Proteomics: Machine learning algorithms analyze over 200 proteins to estimate biological age [32]
These methods help identify reliable aging biomarkers. These are physiological and molecular indicators that show age-related structural or functional degeneration [5].
Animal models that reveal aging mechanisms
Mice, rats, fruit flies (Drosophila melanogaster), and roundworms (C. elegans) remain the classic research models. Their well-known genetics and short lifespans make them ideal [33]. New models also give unique perspectives:
- Long-lived mammals: Naked mole-rats can live beyond 30 years – ten times longer than mice [33]
- Positive outliers: Bats live 3-9.8 times longer than similar-sized mammals despite high metabolic rates [33]
- Accelerated aging models: D-galactose treatment and senescence-accelerated mouse strains show faster aging [34]
Each model helps scientists learn about specific aspects of aging biology.
Meet the geroscientists pioneering aging research
Geroscience studies biological mechanisms of aging and moves forward faster through dedicated researchers and institutions. Yale’s Translational Geroscience Initiative supports cross-disciplinary research on aging blood vessels, inflammation, and immune system changes [35]. Cedars-Sinai’s Center on Translational Geroscience runs clinical trials. They test senolytics and metformin to treat age-related conditions [36].
Resource Links:
- National Institute on Aging: https://www.nia.nih.gov/research
- American Federation for Aging Research: https://www.afar.org
- Buck Institute for Research on Aging: https://www.buckinstitute.org
Conclusion
Our scientific understanding of aging has transformed over time. Research now shows that aging isn’t just a steady decline but happens in distinct biological phases. The most important molecular changes happen around ages 30, 44, and 60 and affect many cellular systems at once.
Groundbreaking research from Stanford Medicine shows how proteins, DNA repair mechanisms, and mitochondrial function go through dramatic changes at these crucial points. The body’s telomeres get shorter more quickly while oxidative stress builds up. These changes create ripple effects throughout our biological systems.
This knowledge reaches way beyond the reach and influence of pure scientific interest. Scientists can now develop targeted treatments for age-related conditions. The research also helps measure biological age more accurately through protein biomarkers and DNA methylation patterns.
Research teams keep learning about these complex aging mechanisms. They use advanced methods like the largest longitudinal study and sophisticated biomarker analysis. Their findings could lead to better ways to stay healthy as we age.
Resource Links:
- National Institute on Aging: https://www.nia.nih.gov/health/aging-research
- American Federation for Aging Research: https://www.afar.org/research
- Stanford Center on Longevity: https://longevity.stanford.edu/research
FAQs
At what age do significant biological changes associated with aging begin?
Significant biological changes associated with aging start as early as age 30. This is when cellular processes like telomere shortening, mitochondrial efficiency decline, and DNA repair mechanism slowdown begin to accelerate. However, aging isn’t a steady process; research shows distinct transition periods around ages 44 and 60 where more dramatic molecular shifts occur.
How does aging affect our body at the molecular level?
Aging affects our body at the molecular level in various ways. These include telomere shortening, decreased mitochondrial function, accumulation of DNA damage, protein folding errors, and increased oxidative stress. These changes impact cellular function and contribute to the overall aging process.
What are some key biomarkers scientists use to measure biological age?
Scientists use several biomarkers to measure biological age, including DNA methylation patterns, telomere length, and specific protein levels in the blood. Advanced techniques like proteomics can analyze over 200 proteins to estimate biological age more accurately than chronological age alone.
How do protein changes contribute to the aging process?
Protein changes significantly contribute to aging. As we age, there’s an increase in protein folding errors and a decline in the efficiency of the proteostasis network, which maintains protein balance. This leads to the accumulation of misfolded proteins, which can interfere with cellular functions and contribute to age-related decline.
Are there ways to slow down the biological aging process?
While aging is a natural process, research suggests certain lifestyle factors may help slow biological aging. These include regular exercise, a balanced diet rich in antioxidants, adequate sleep, stress management, and avoiding harmful habits like smoking. However, the effectiveness of interventions can vary among individuals, and more research is needed in this area.
References
[1] – https://pmc.ncbi.nlm.nih.gov/articles/PMC9909609/
[2] – https://www.frontiersin.org/journals/aging-neuroscience/articles/10.3389/fnagi.2022.827900/full
[3] – https://www.theguardian.com/science/article/2024/aug/14/scientists-find-humans-age-dramatically-in-two-bursts-at-44-then-60-aging-not-slow-and-steady
[4] – https://www.bannerhealth.com/healthcareblog/teach-me/understanding-alcohols-impact-on-your-body-as-you-age
[5] – https://pmc.ncbi.nlm.nih.gov/articles/PMC11081160/
[6] – https://jamanetwork.com/journals/jamanetworkopen/fullarticle/2765370
[7] – https://pubmed.ncbi.nlm.nih.gov/33552142/
[8] – https://www.frontiersin.org/journals/genetics/articles/10.3389/fgene.2020.630186/full
[9] – https://www.nature.com/articles/s41467-021-24956-2
[10] – https://www.frontiersin.org/journals/physiology/articles/10.3389/fphys.2024.1384966/full
[11] – https://www.aging-us.com/article/101120/text
[12] – https://pmc.ncbi.nlm.nih.gov/articles/PMC2190694/
[13] – https://pmc.ncbi.nlm.nih.gov/articles/PMC5748716/
[14] – https://pmc.ncbi.nlm.nih.gov/articles/PMC3901353/
[15] – https://www.synbiobeta.com/read/stanford-research-unveils-molecular-transformations-in-your-40s-and-60s
[16] – https://www.webmd.com/diet/features/fighting-40s-flab
[17] – https://www.niaaa.nih.gov/alcohols-effects-health/aging-and-alcohol
[18] – https://www.nature.com/articles/s41392-023-01378-8
[19] – https://www.nytimes.com/2024/10/27/opinion/body-muscles-40s-aging.html
[20] – https://pubmed.ncbi.nlm.nih.gov/30733602/
[21] – https://pmc.ncbi.nlm.nih.gov/articles/PMC3402352/
[22] – https://news.emory.edu/stories/2014/01/aging_brains_need_chaperone_proteins/index.html
[23] – https://elifesciences.org/articles/48240
[24] – https://www.sciencedirect.com/science/article/abs/pii/S0531556503001852
[25] – https://www.statnews.com/2019/12/05/scientists-develop-a-clock-that-can-measure-biological-age-based-on-blood-proteins/
[26] – https://hms.harvard.edu/news/gaging-biological-age-disease-risk-proteomic-clock
[27] – https://www.nature.com/articles/s41591-024-03164-7
[28] – https://www.nature.com/articles/s43587-024-00692-2
[29] – https://www.news-medical.net/news/20240816/Scientists-discover-key-age-related-biological-shifts-at-40-and-60.aspx
[30] – https://www.genomeweb.com/sequencing/multiomics-study-marks-two-periods-significant-molecular-change-during-aging
[31] – https://pmc.ncbi.nlm.nih.gov/articles/PMC2423215/
[32] – https://www.aging-us.com/article/101414/text
[33] – https://www.frontiersin.org/journals/molecular-biosciences/articles/10.3389/fmolb.2021.660959/full
[34] – https://pmc.ncbi.nlm.nih.gov/articles/PMC9102583/
[35] – https://medicine.yale.edu/news/yale-medicine-magazine/article/geroscience/
[36] – https://www.cedars-sinai.org/discoveries/study-of-aging-pioneered-at-cedars-sinai.html